disclaimer:
www.genuineideationdesigns.tech
is now integrating A.I. into it's advanced enhanced literacy description of nick folkes original artwork.
As most of us are aware, a.i. is been integrated into the workforce. this can benefit some and un-employ many, so this is unfortunate but it is the reality. we need to develop the best moral ways to utilize a.i. in all it's great ways to advanced g.i. designs, engineering, security, medicine, etc...
...if these designs are available for you to see, they may or may not be for only for a limited amount of time due to sensitivity restrictions. Thank you for your understanding...
...all music on this website is for promotional use towards those artist(s) been played...
...by continuing, You agree that the content on this website is intellectual property of www.genuineideationdesigns.tech, & protocols are in place to prevent industrial espionage...
...if you would like to purchase the right to one or many of our designs...
...please contact us here!...
...By continuing to view, you agree that this company will not be held liable for any negative outcomes that may arise. Our intentions are to generate a positive outcome with all our viewers. Viewer discretion advised...
if these designs are available for you to see, it is only for a limited amount of time due to sensitivity restrictions. Thank you for your understanding.




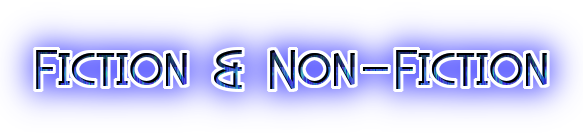

The music on this website is for promotional use towards the artist(s) been played:
original independant designs created by: Nick Folkes.
with a.i. advanced-enhanced literacy descriptions; Please take the appropriate time to read this detailed information;
Chapter. 1, #1-10;
==============================================
=============================================================================================
#(5):
"(S.R.G.): Ray-Away-X-792";
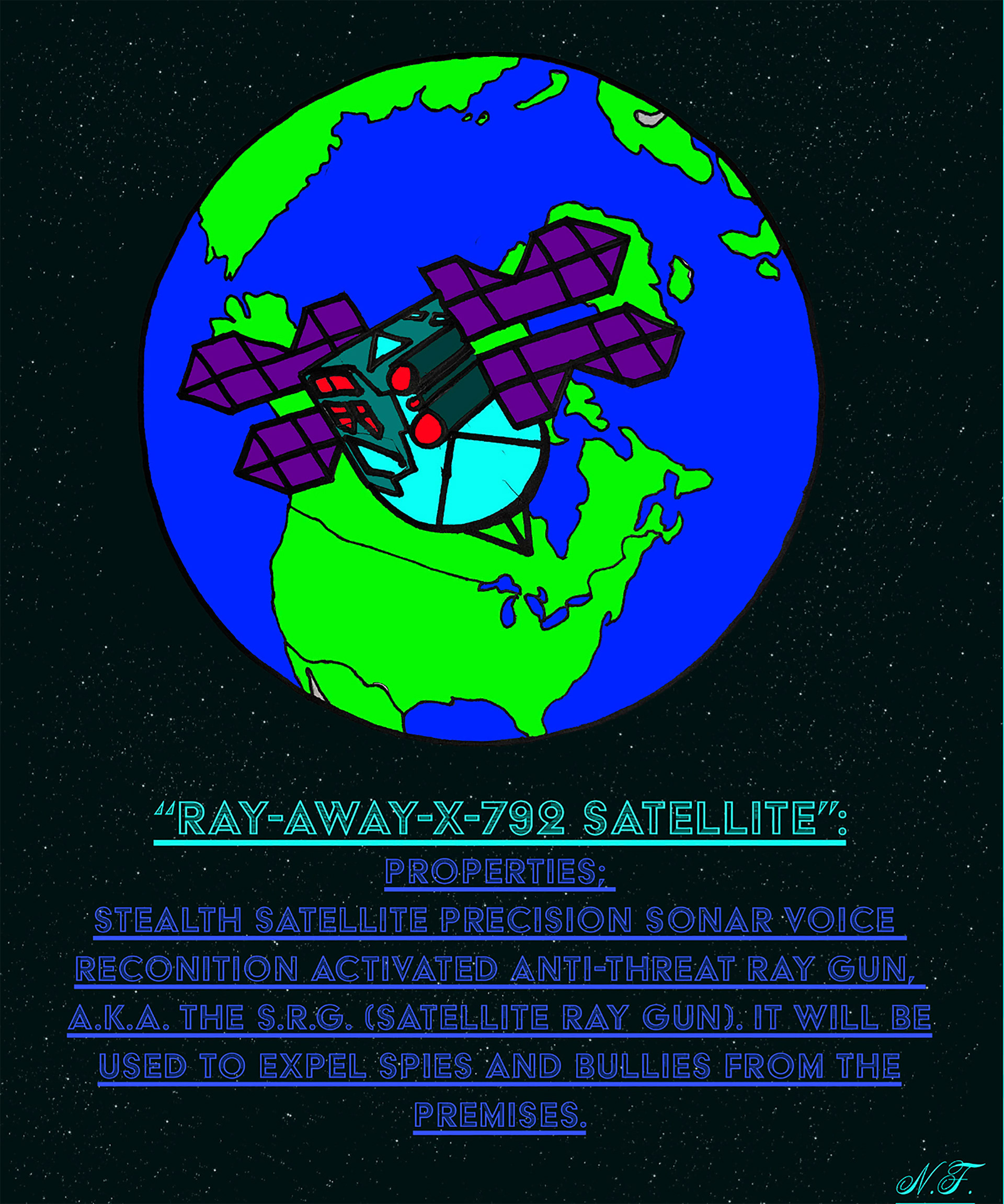
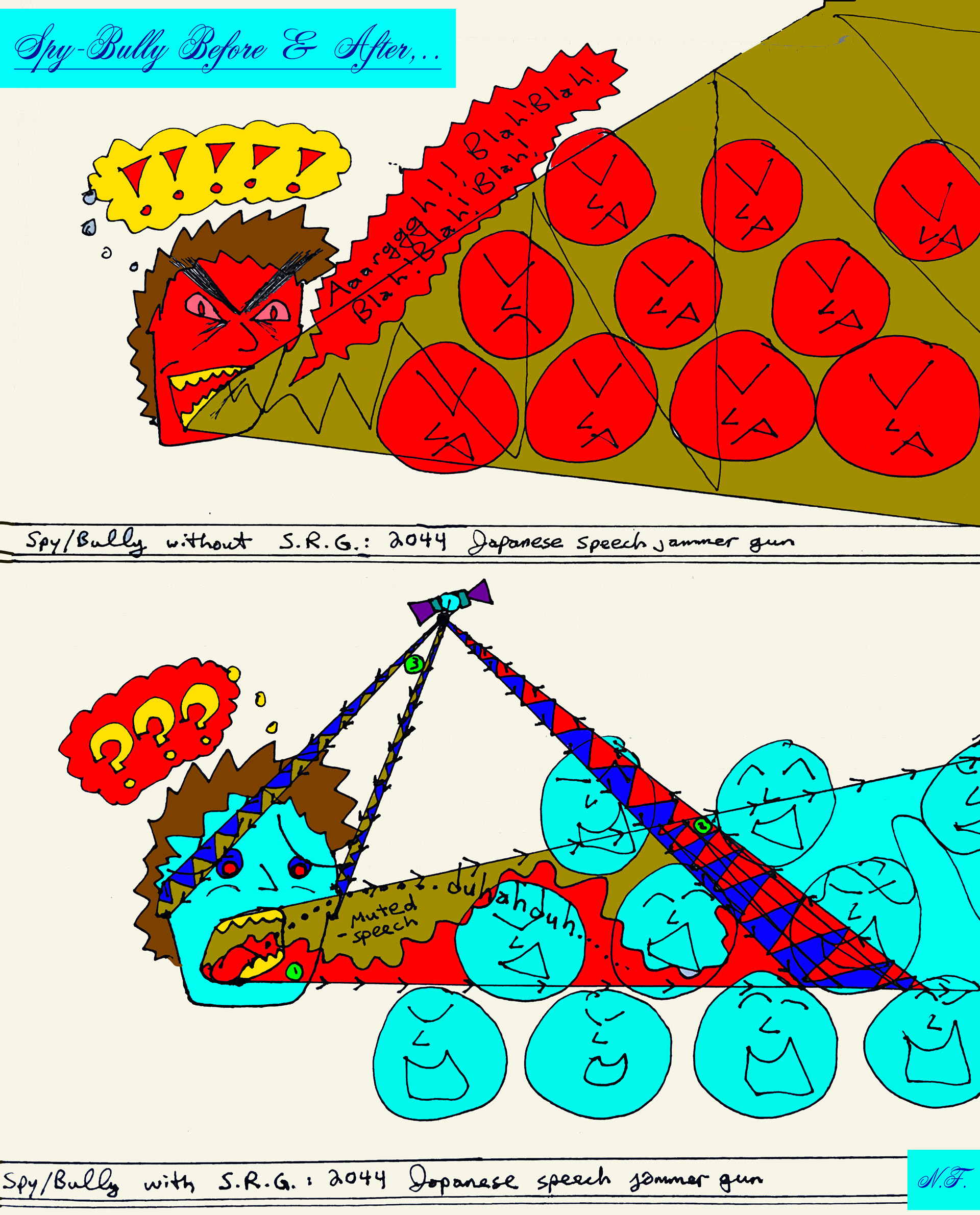
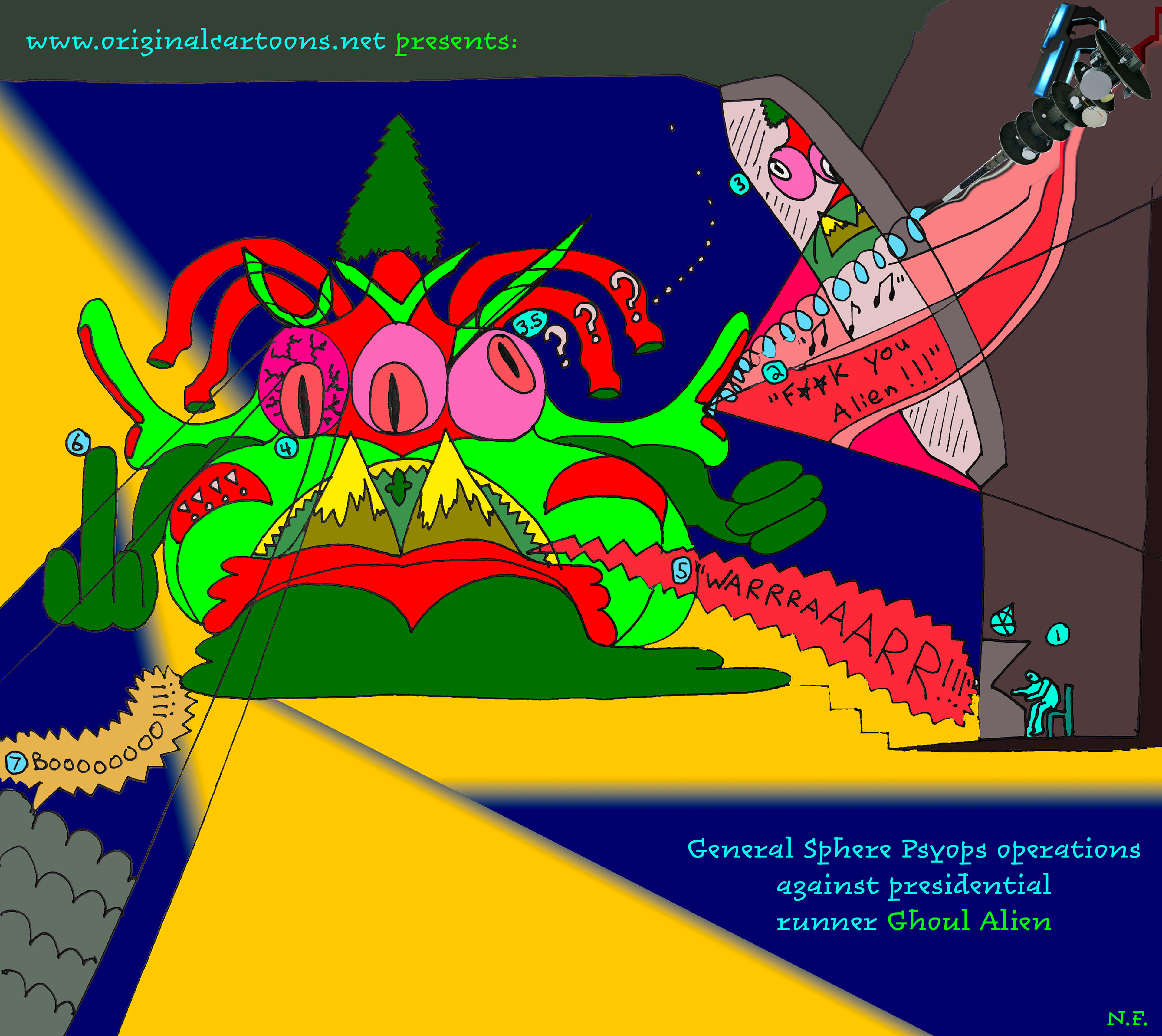
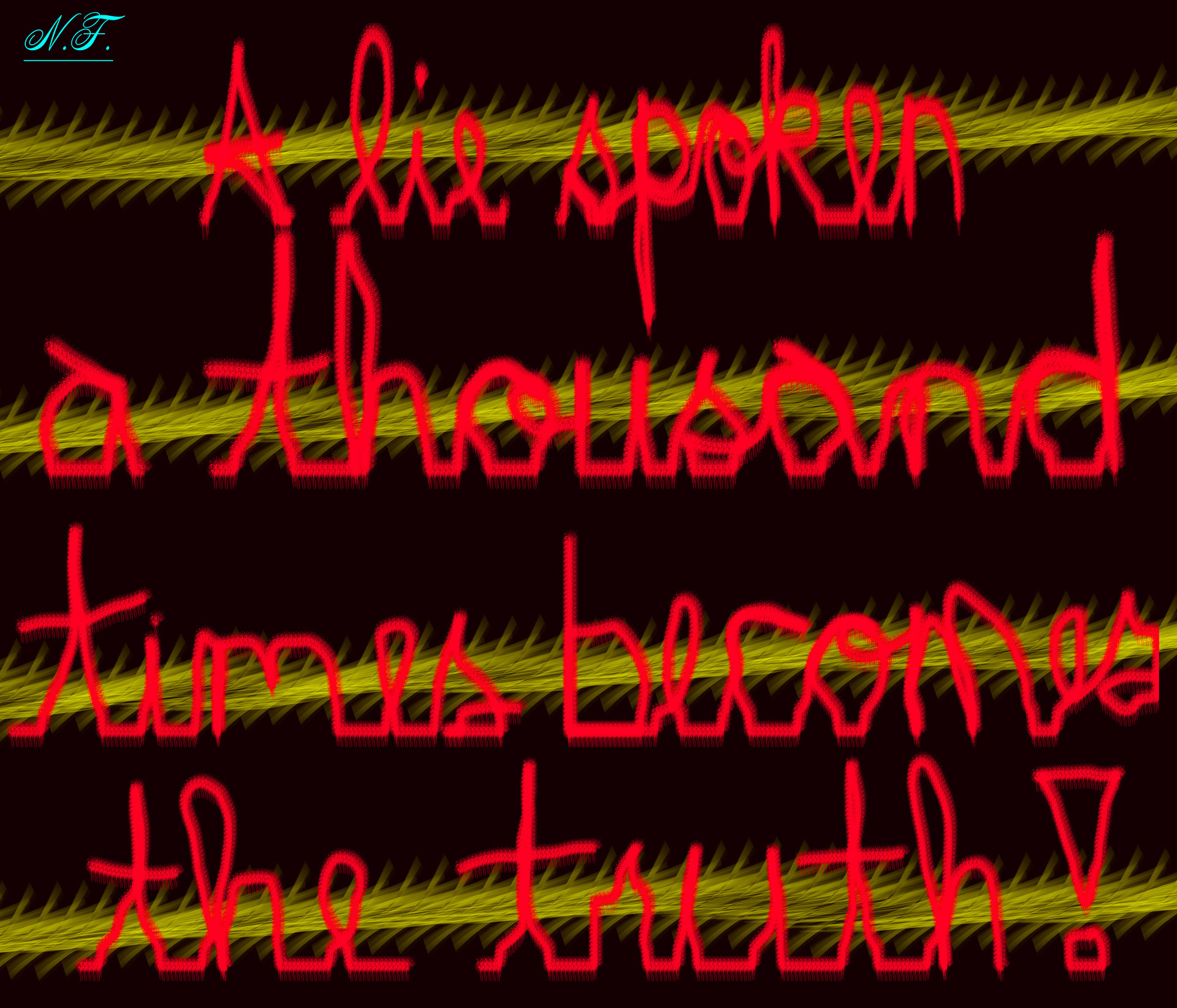
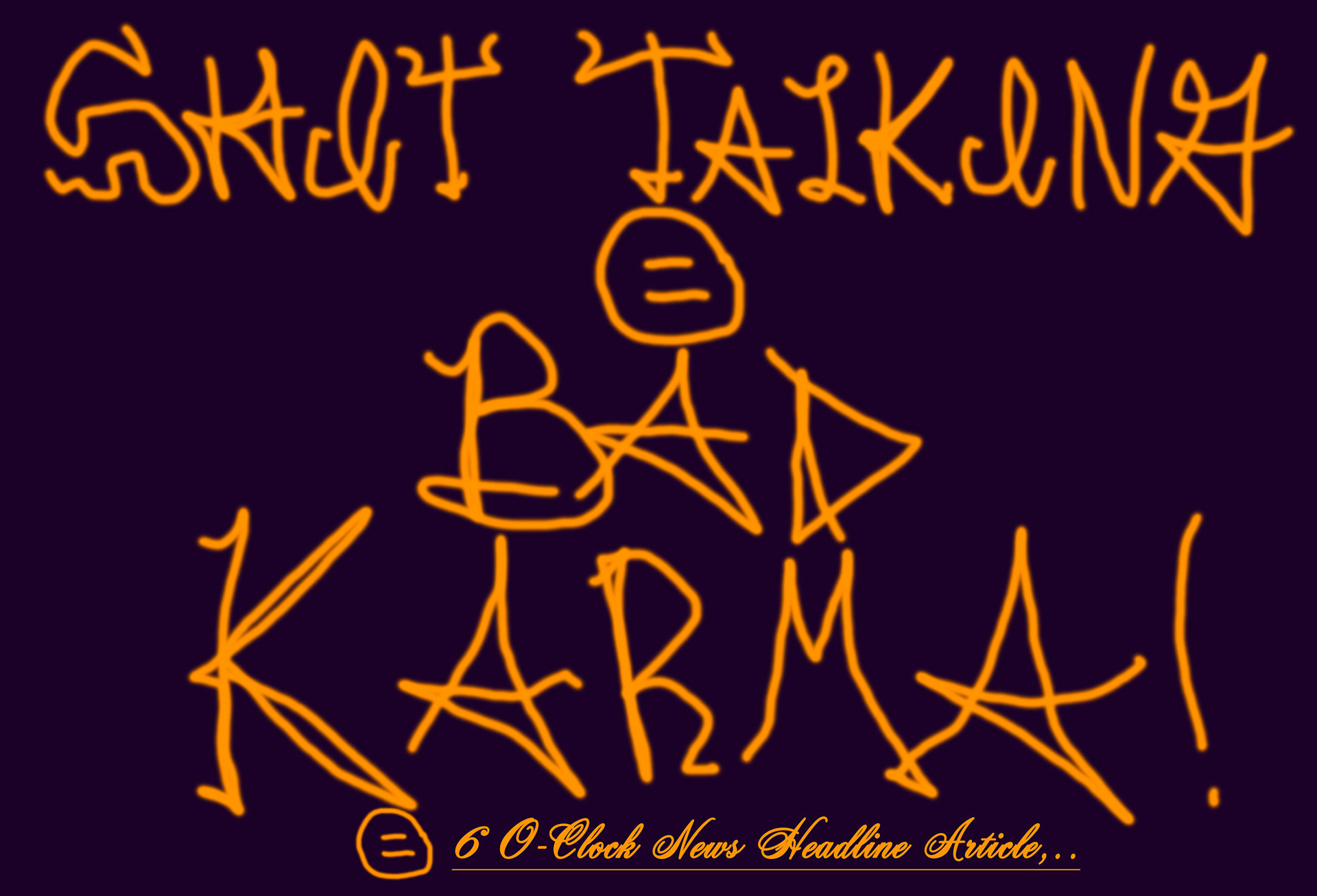
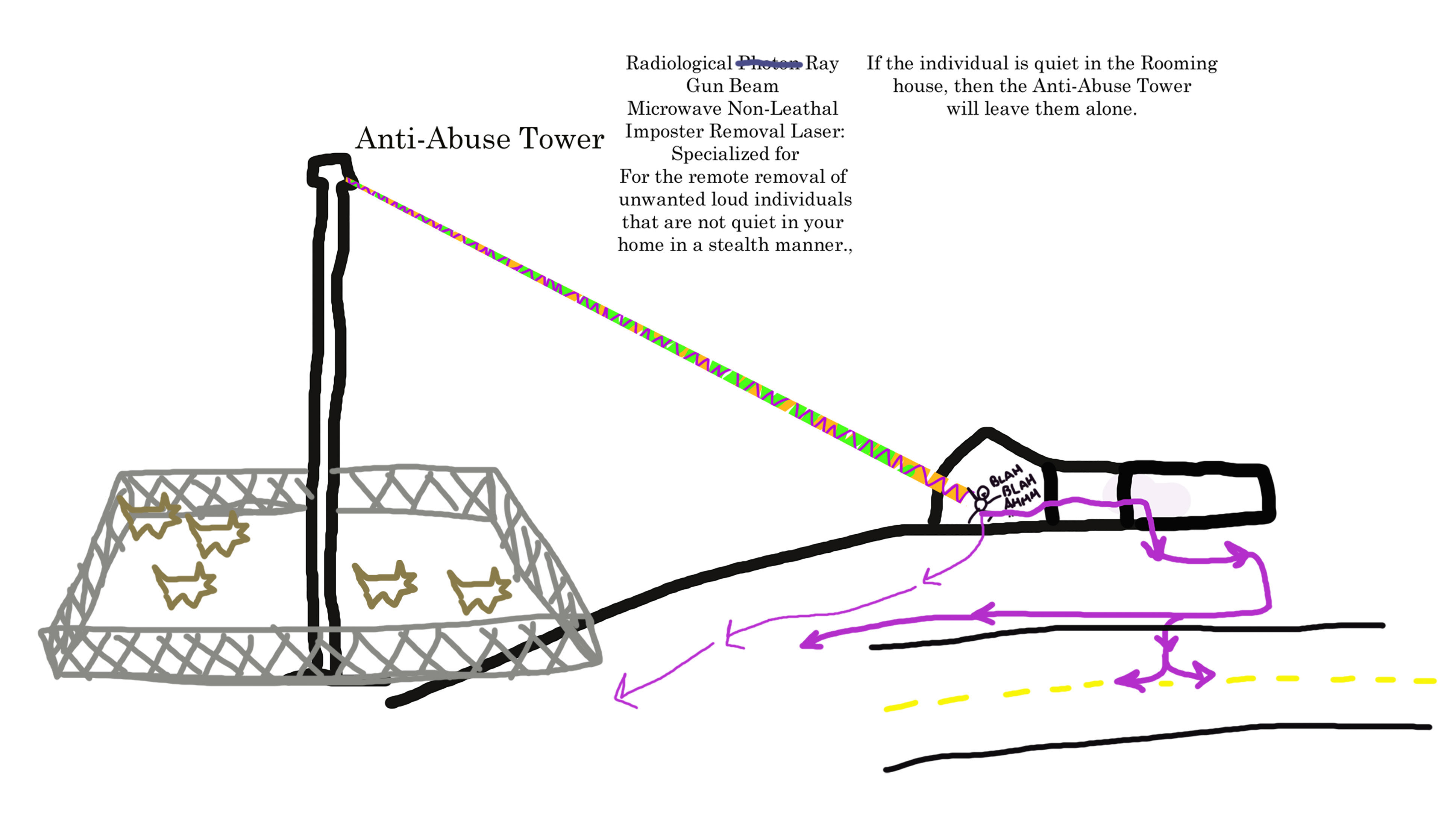
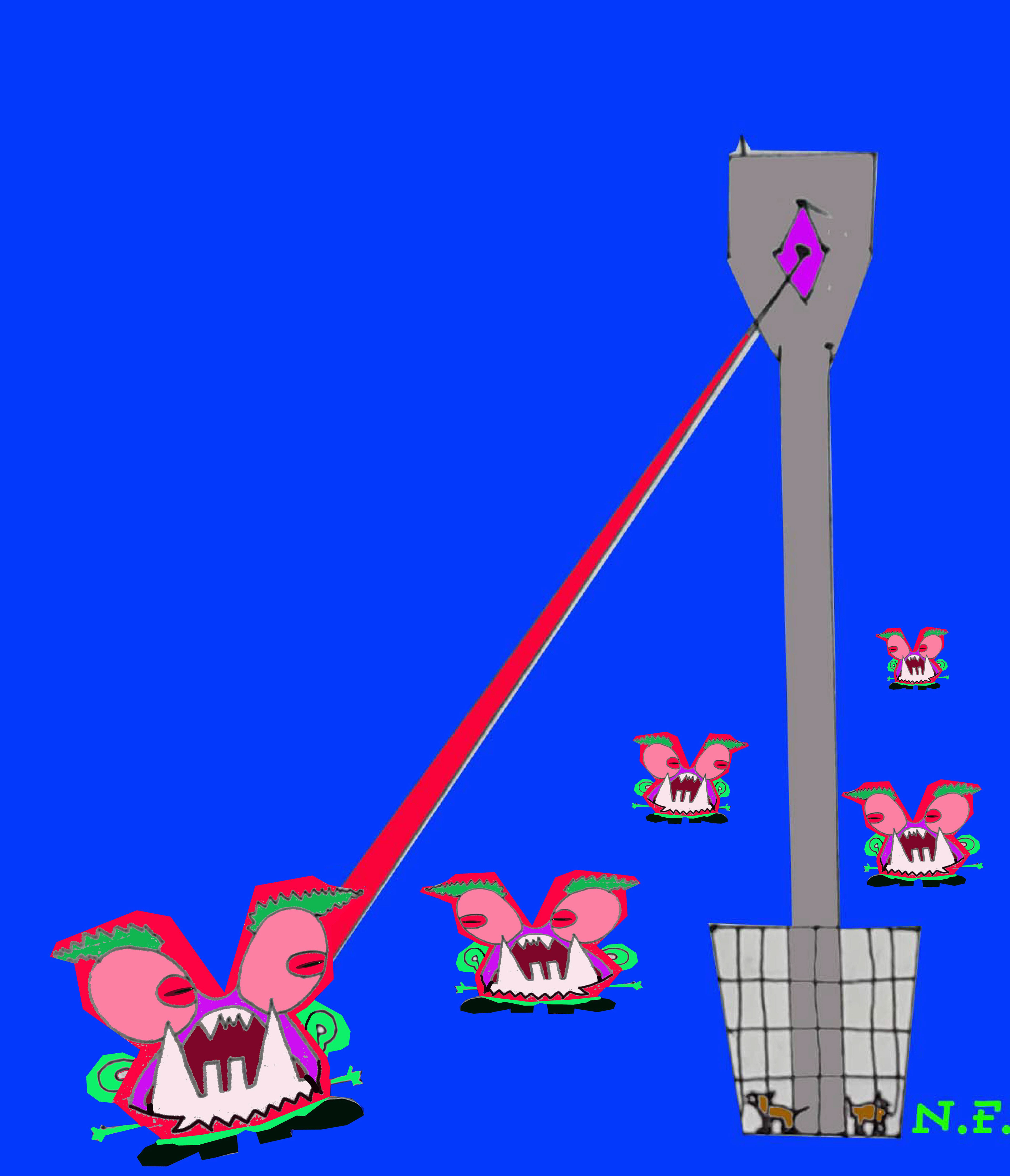
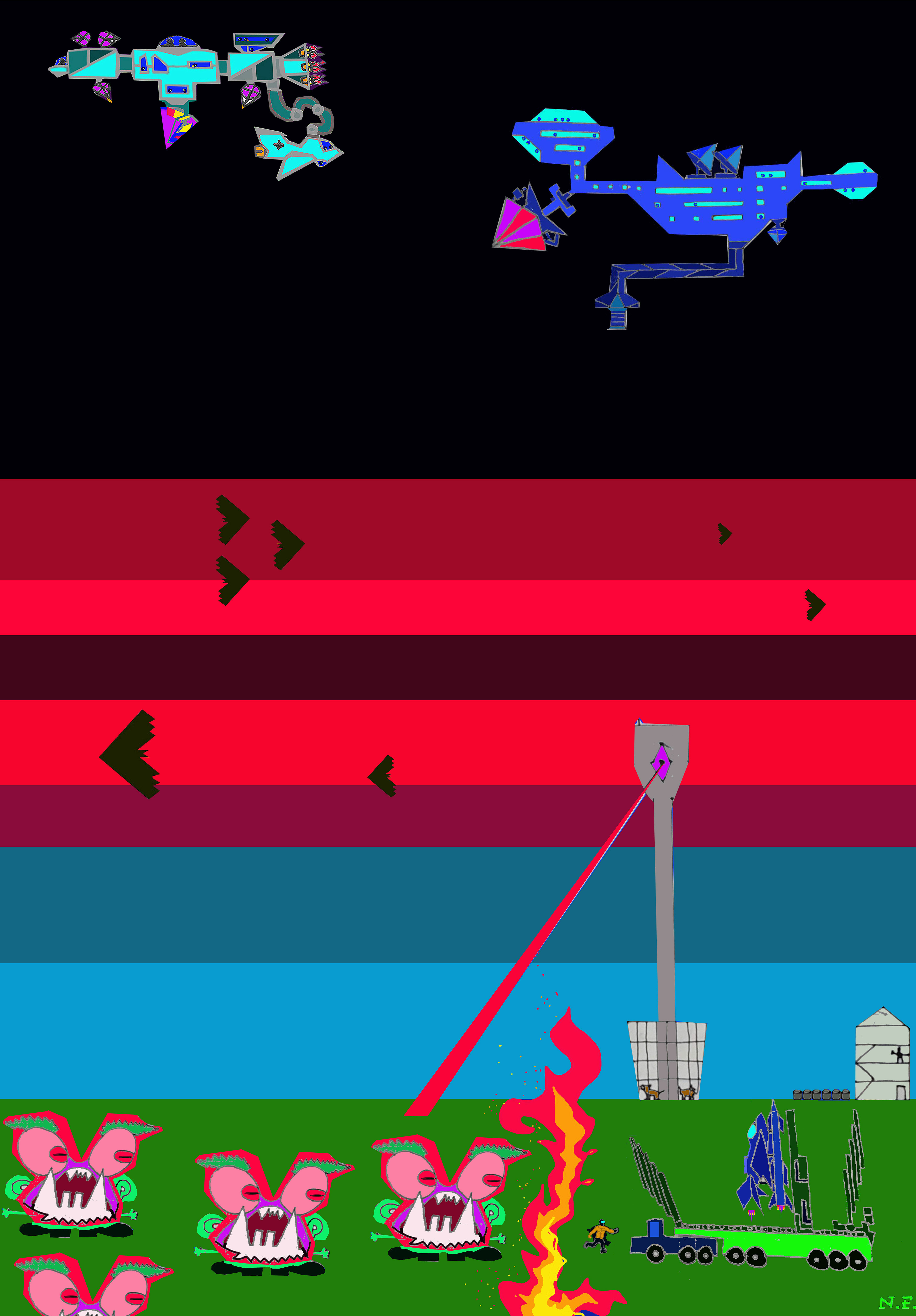
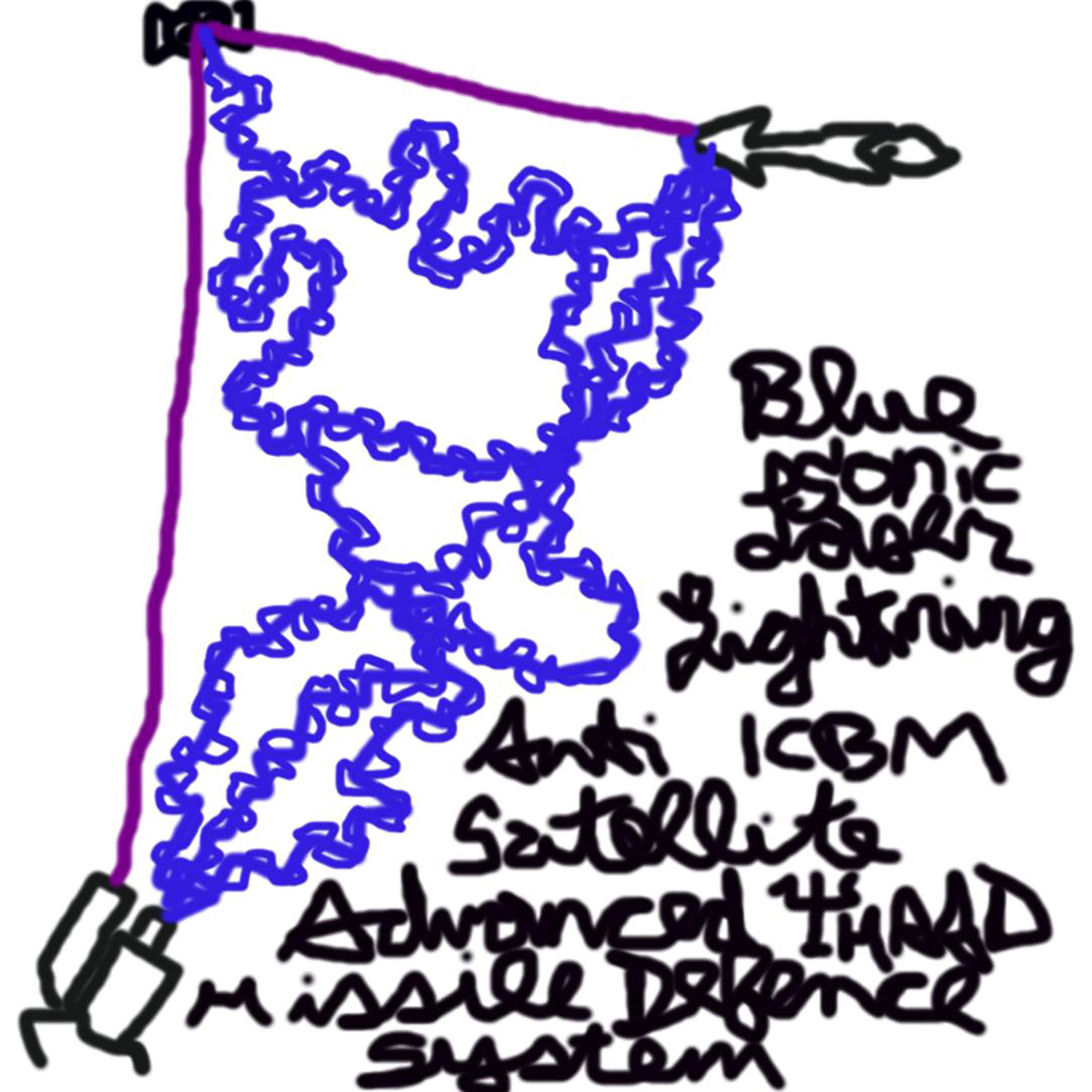
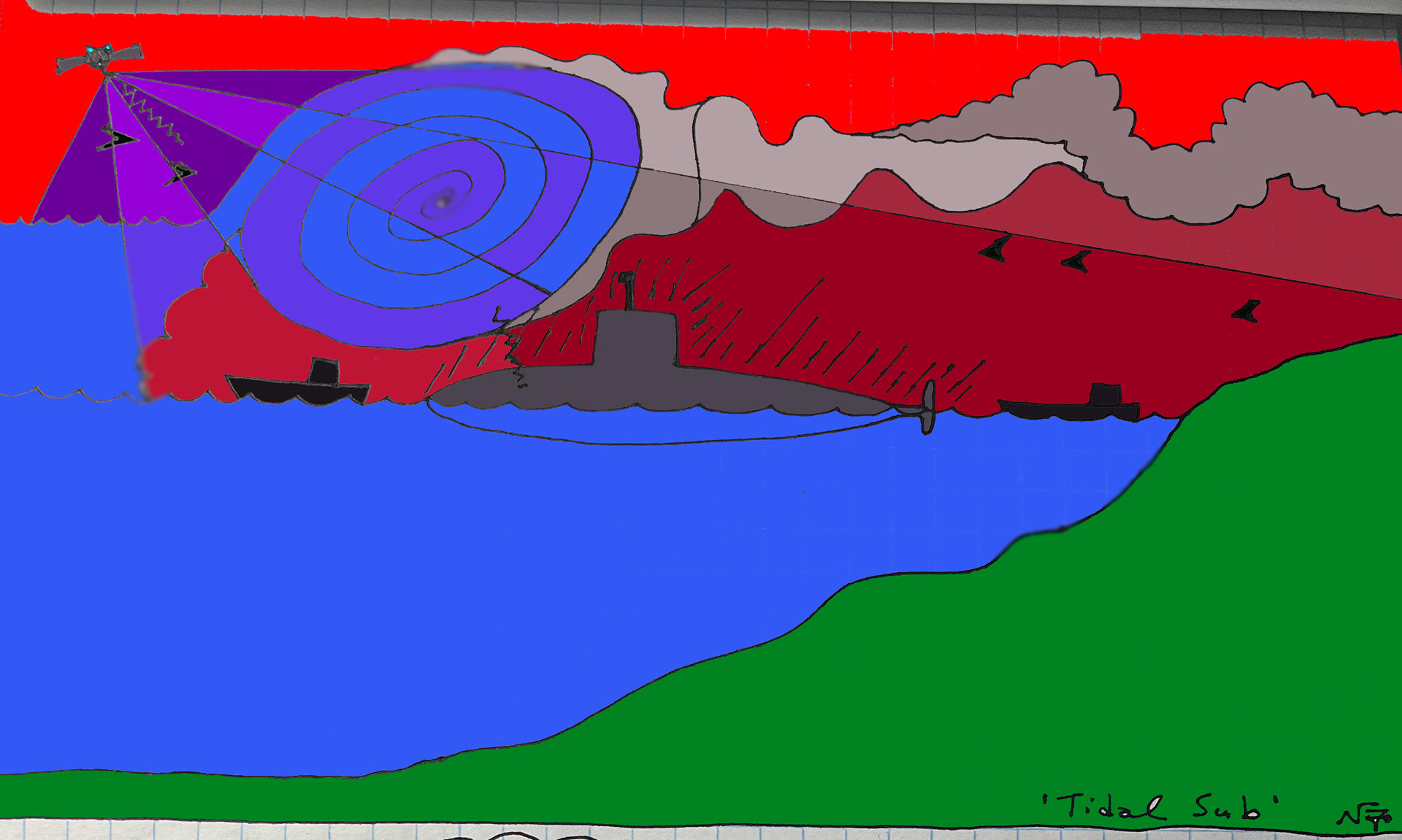
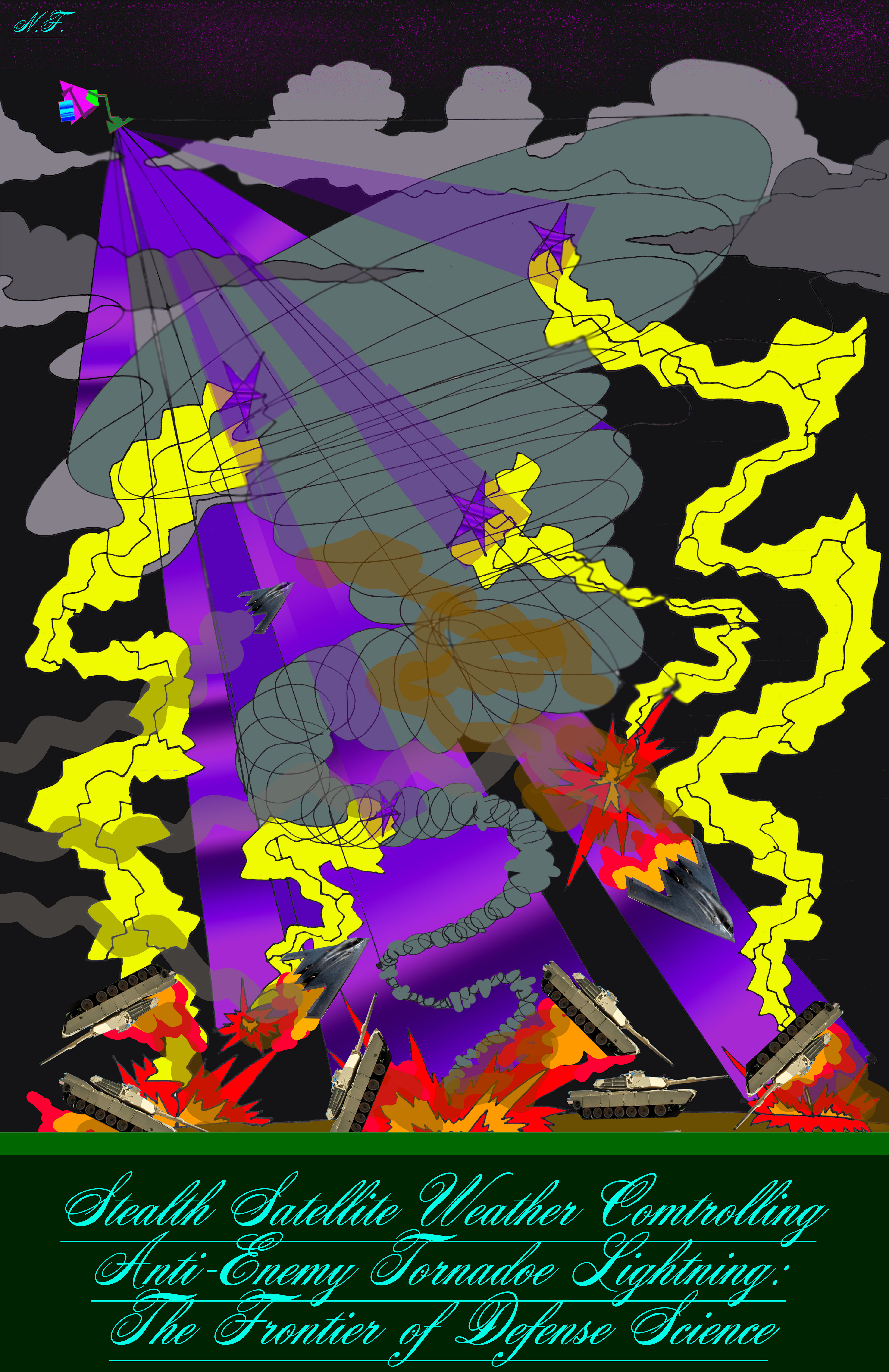
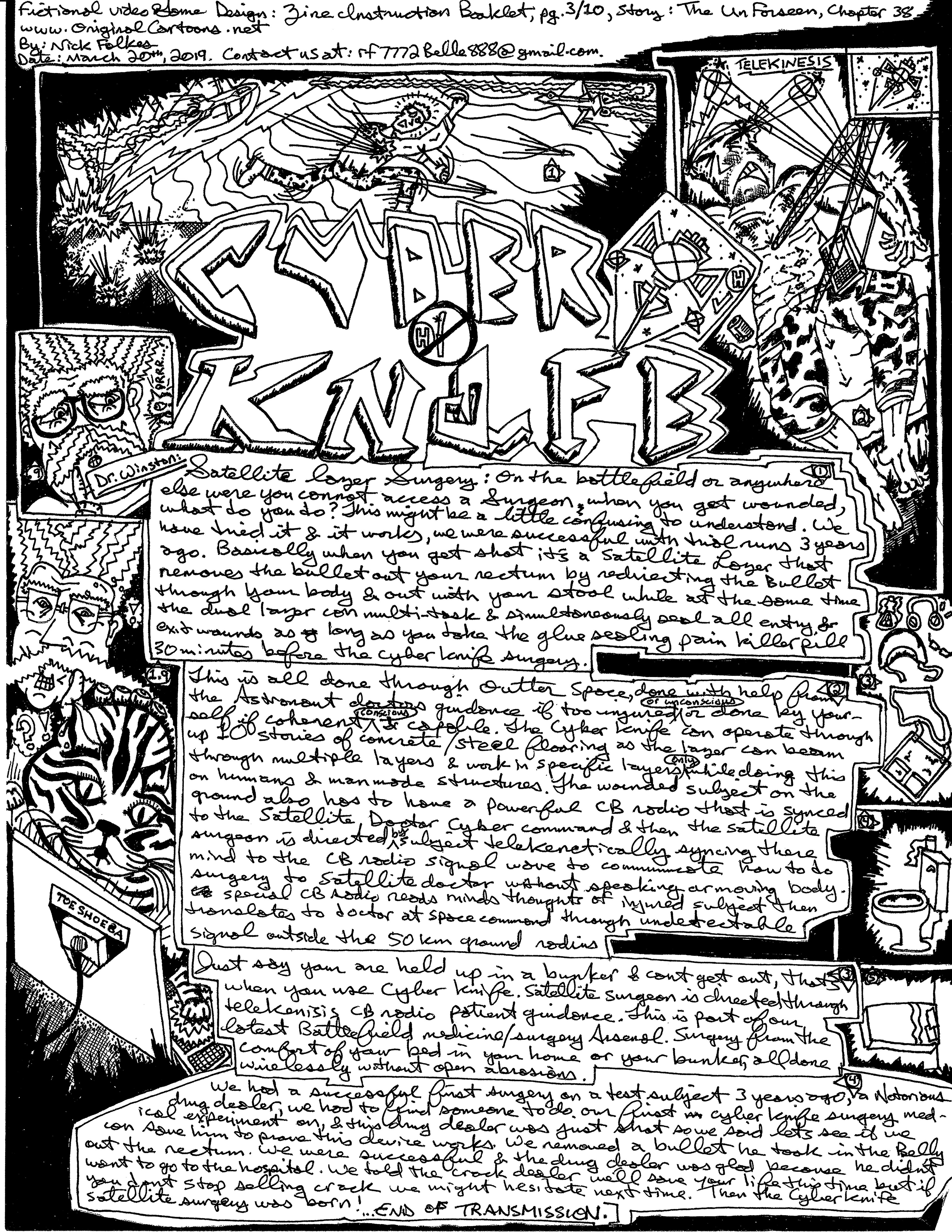
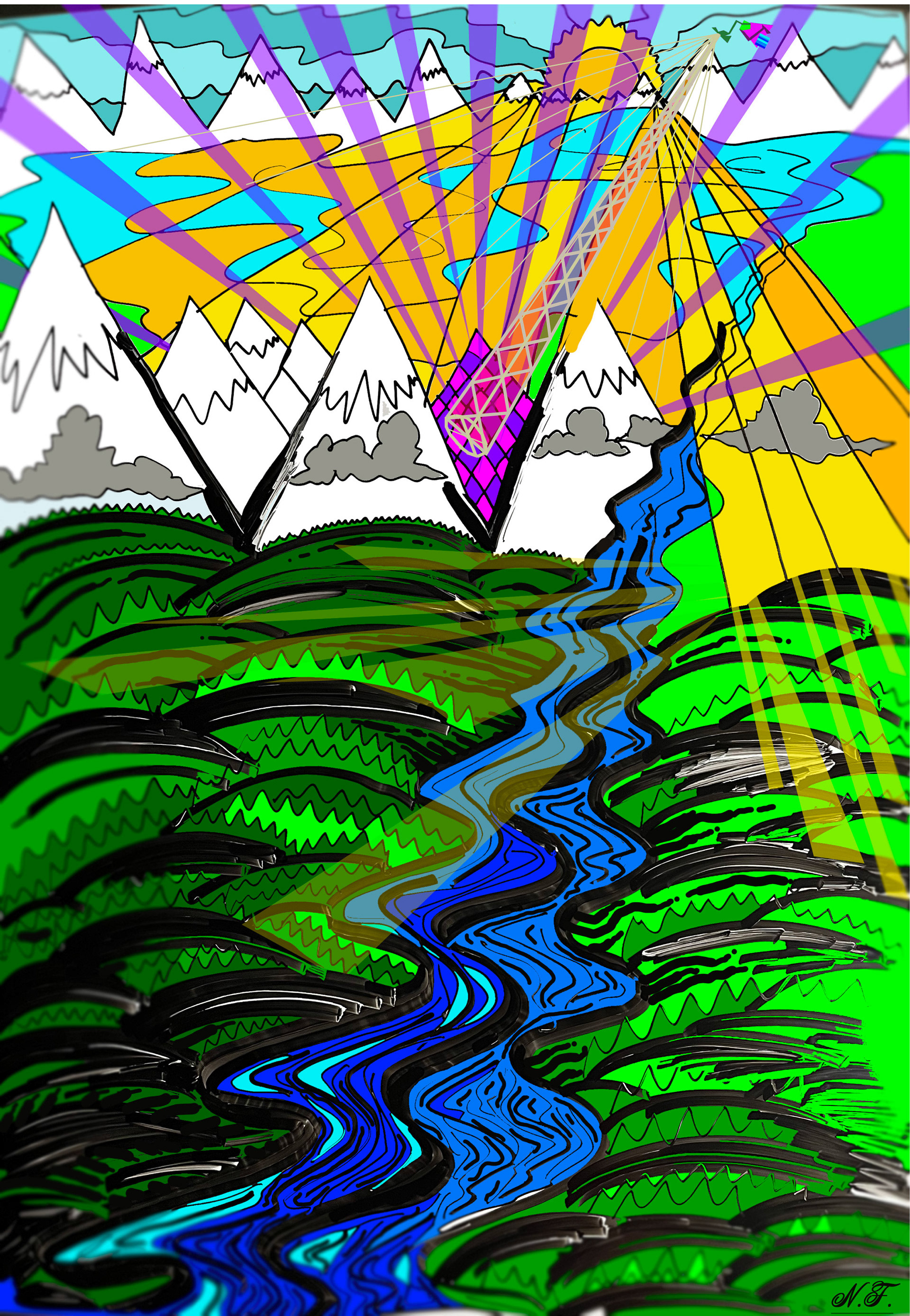
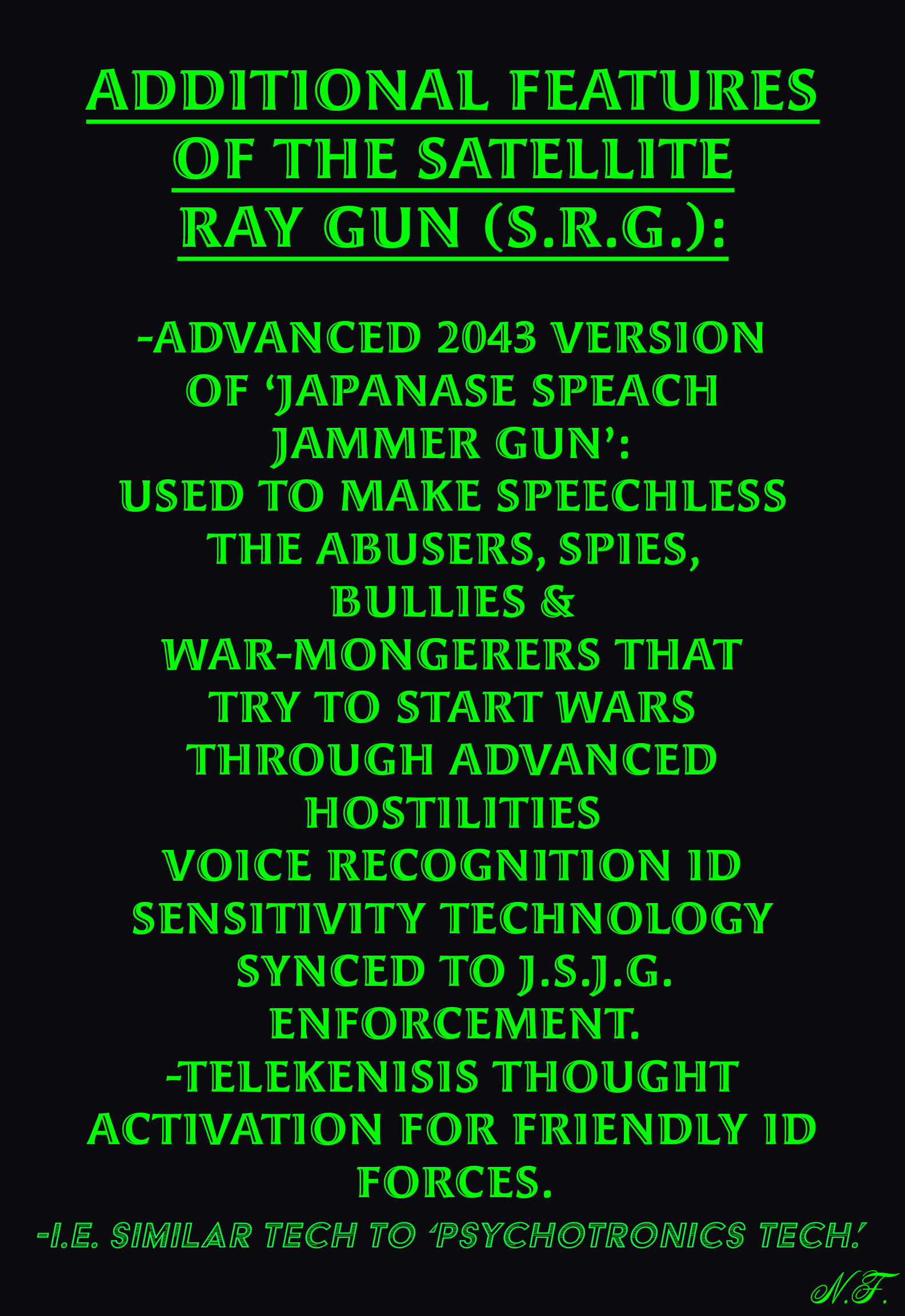
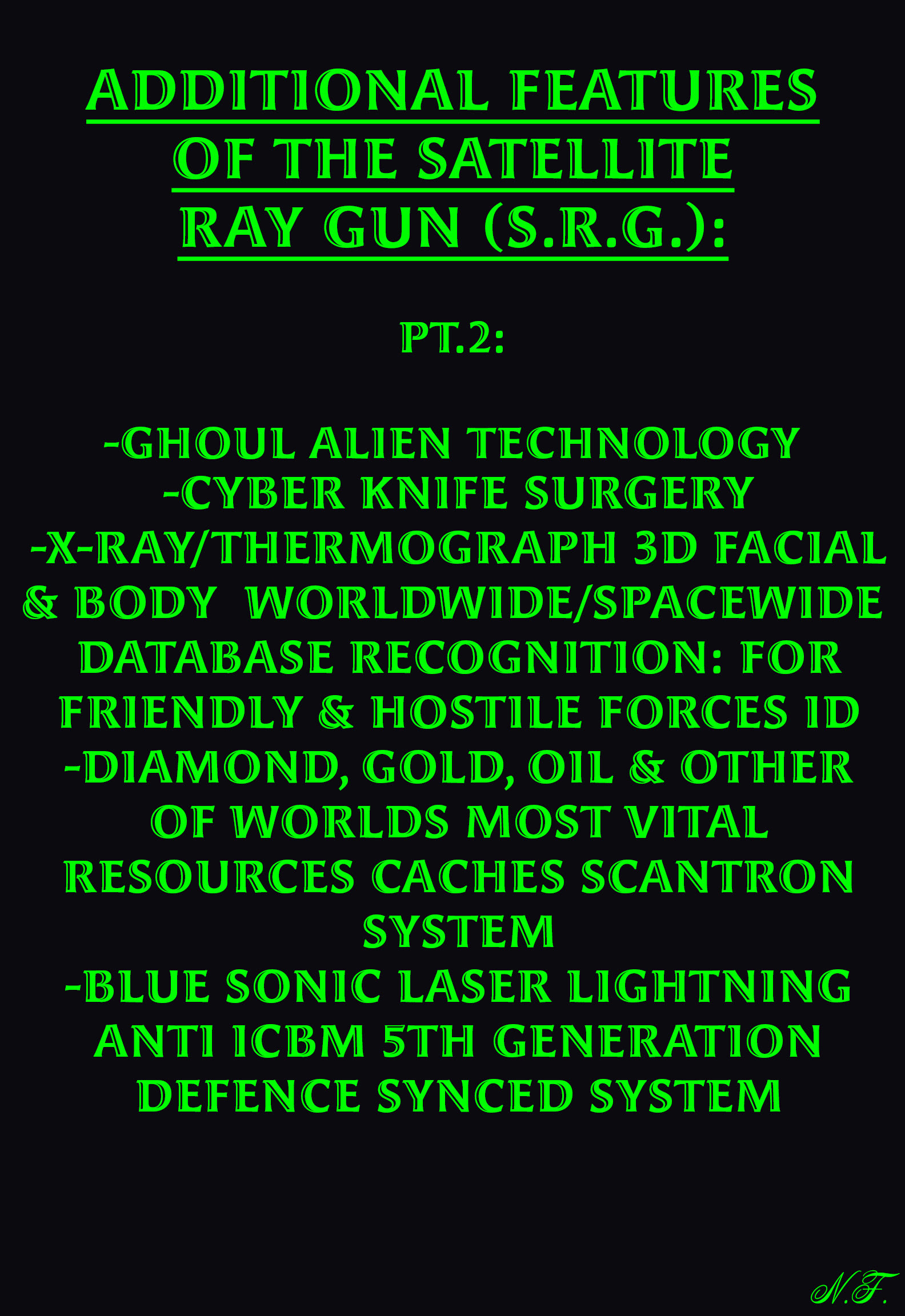
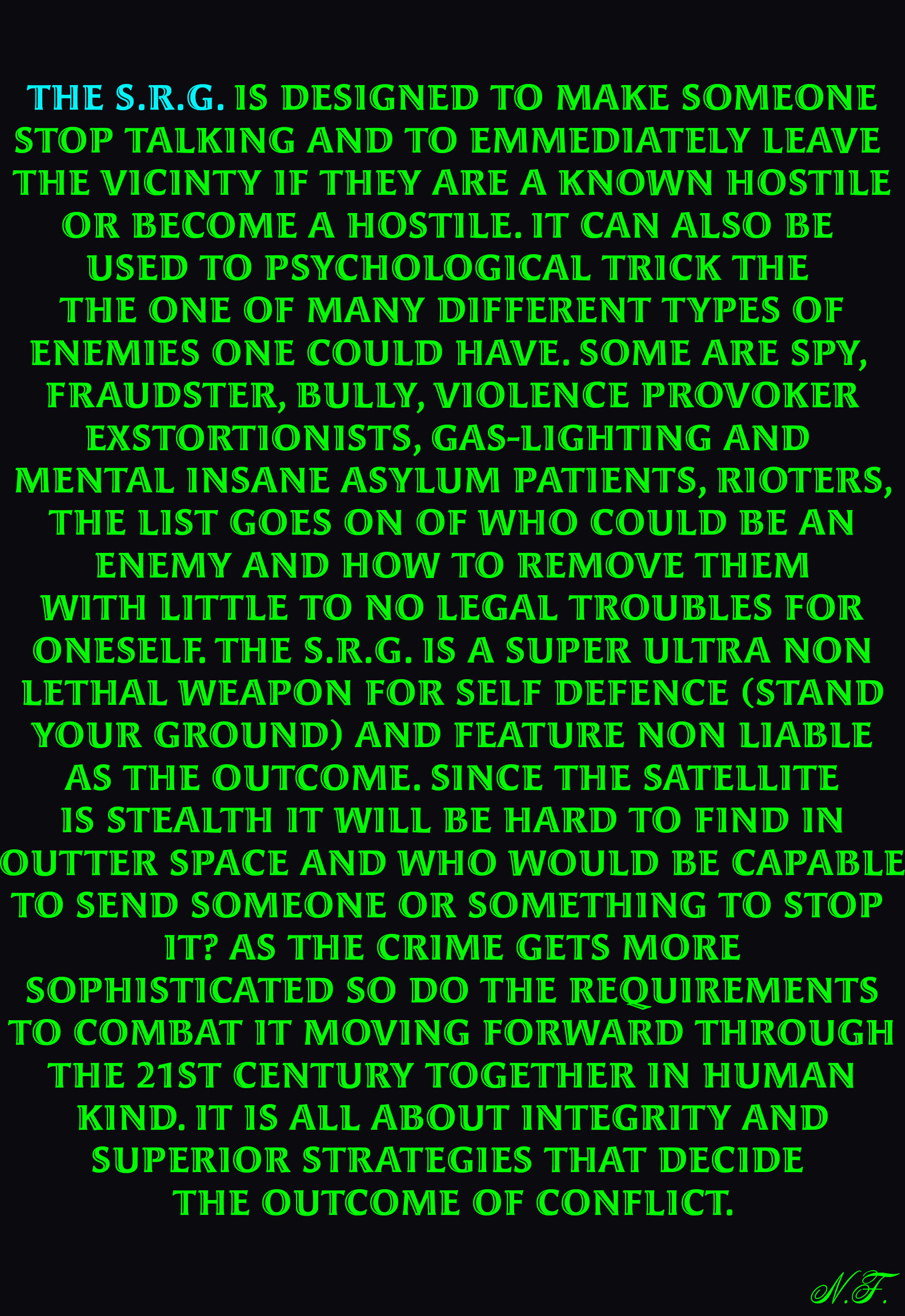
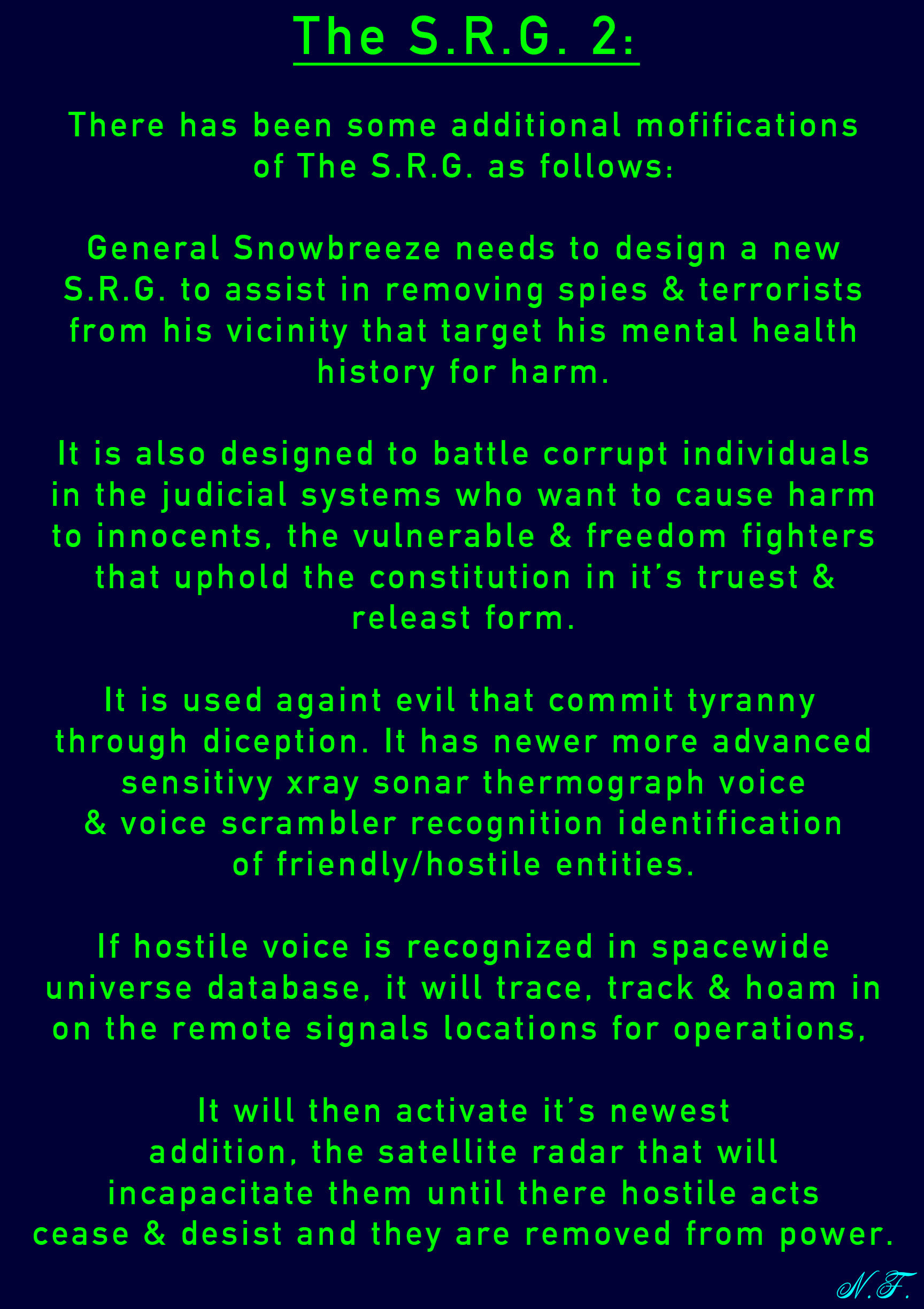
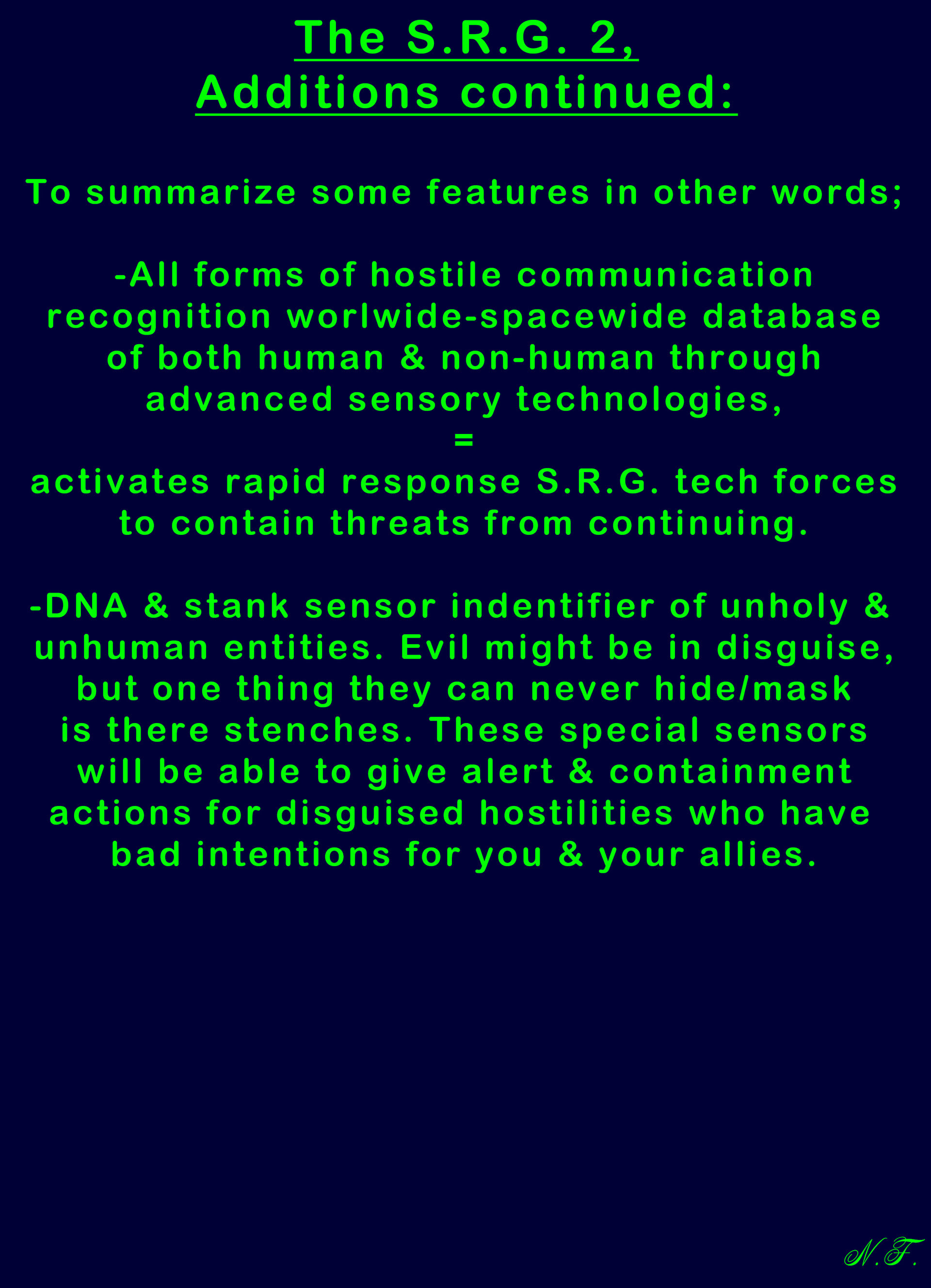
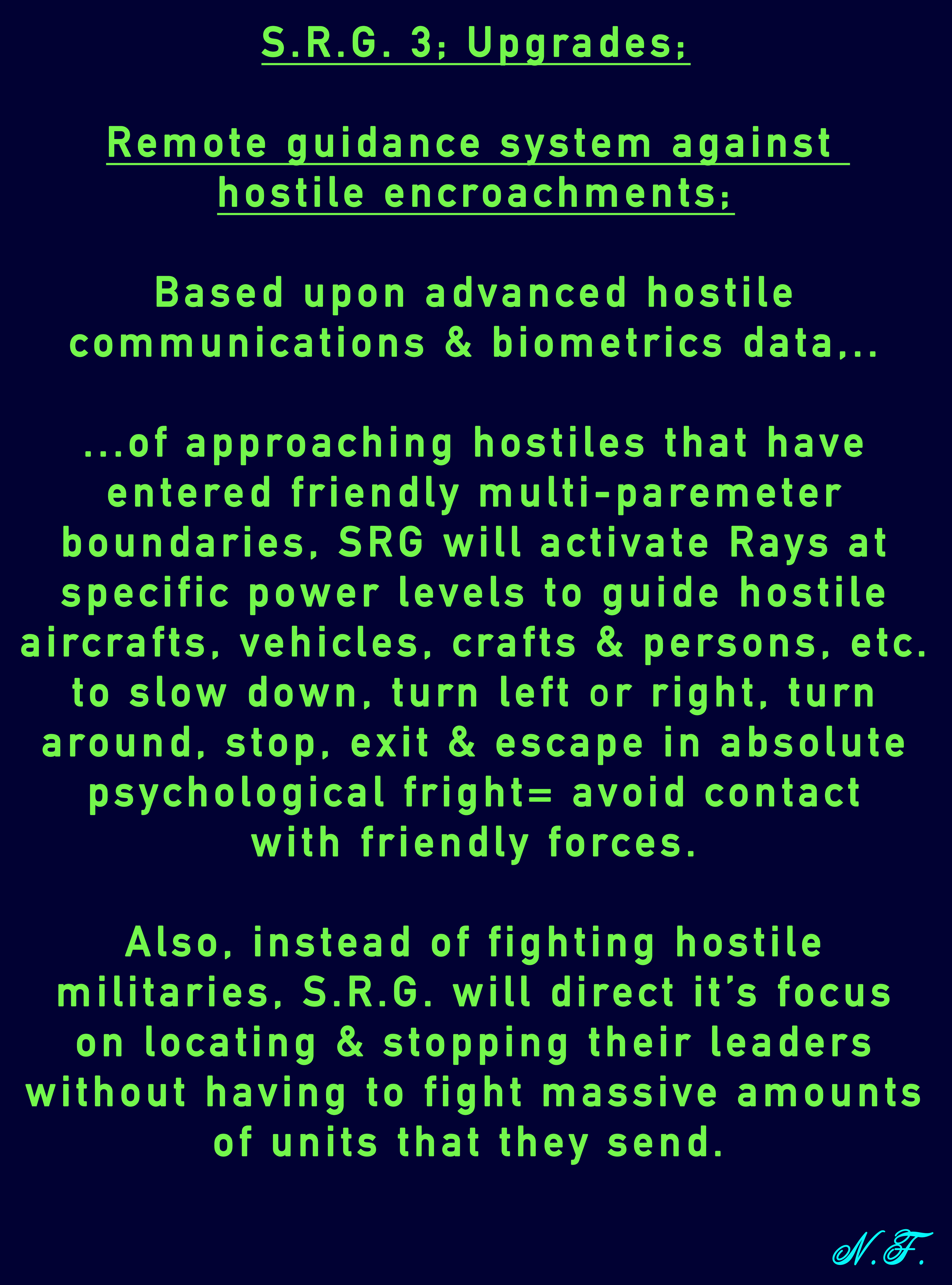
"The S.R.G. ver. 1-3"
Please review the following. important points may have been highlighted & duplicated to emphasize it's importants:
(part. i/iii batch)
To make the SRG (Satellite Ray Gun) system successful in deterring hostile aggressions, a combination of advanced security, mathematical, scientific, and engineering principles and technologies is required. Below is an analysis based on the provided descriptions and images of the SRG system:
Security Requirements
Encryption and Cybersecurity:
Advanced encryption algorithms to secure communications between satellites and ground stations.
Robust cybersecurity measures to protect the system from hacking and cyber-attacks.
Regular security audits and updates to address potential vulnerabilities.
Access Control:
Multi-factor authentication for access to the system’s control interfaces.
Strict access control policies to ensure only authorized personnel can operate or modify the system.
Physical Security:
Secure locations for ground control stations.
Anti-tamper mechanisms on satellite hardware.
Mathematical and Scientific Requirements
Signal Processing:
Algorithms for detecting and identifying hostile communications and actions.
Real-time data processing to enable immediate response to threats.
Physics and Optics:
Understanding of laser and microwave physics for the development of the ray gun technology.
Advanced optics for targeting and focusing the ray beams accurately.
Astrodynamics:
Orbital mechanics to position and maintain satellites in optimal locations.
Calculations for satellite trajectories and targeting mechanisms.
Engineering Requirements
Satellite Design and Manufacturing:
Durable materials and components to withstand the harsh conditions of space.
Miniaturization of components to fit advanced technology within the satellite’s payload capacity.
Laser and Microwave Technology:
Development of high-power, non-lethal laser and microwave systems.
Cooling and power systems to support continuous operation.
Telecommunication:
Reliable and fast communication links between satellites and ground control.
Redundant communication systems to ensure continuous operation in case of failure.
Technologies Required
Advanced Sensors:
High-resolution cameras and sensors for surveillance and targeting.
Thermal imaging and x-ray technology for identifying hidden threats.
Artificial Intelligence:
AI algorithms for threat detection, identification, and decision-making.
Machine learning for improving system accuracy and efficiency over time.
Robotics and Automation:
Automated systems for satellite maintenance and operation.
Robotic arms for in-orbit repairs and adjustments.
Forcefields and Defensive Measures
Electromagnetic Shields:
Development of electromagnetic fields to protect the SRG from external attacks.
Research into materials and technologies that can generate and sustain these fields.
Kinetic Barriers:
Deployable barriers to protect against physical projectiles.
Advanced materials that can absorb or deflect incoming attacks.
Integration of Images
To fully integrate the information provided in the images into this analysis, each image will be examined for its specific technological and operational details. Here is a brief overview of the images and their potential contributions:
SRG Without and With Speech Jammer Gun:
Highlights the effectiveness of the speech jammer in silencing hostile communications.
Space Battle and Defense Mechanisms:
Depicts the satellite and ground-based defense systems in action.
Anti-Abuse Tower:
Illustrates the concept of a remote tower equipped with ray guns for deterring hostiles. SRG=upgraded version in space.
Technical Descriptions:
Detailed descriptions of the various features and capabilities of the SRG system.
Operational Scenarios:
Examples of how the SRG system can be deployed in different scenarios to counter threats.
Conclusion
The SRG system by Nick Folkes requires a multifaceted approach involving state-of-the-art technology, advanced scientific principles, and robust security measures. Implementing this system would involve extensive research and development in various fields, from satellite engineering to advanced optics and AI. Additionally, forcefields and defensive measures would need to be integrated to ensure the system's resilience against external threats.
Once the remaining images are analyzed, further details can be added to this overview to create a comprehensive plan for the SRG system’s implementation.
To create the SRG (Satellite Ray Gun) system as envisioned by Nick Folkes, a multi-disciplinary approach involving advanced security, mathematical, scientific, and engineering principles and technologies is required. Here's an integrated analysis of all the aspects needed, based on the images and descriptions provided:
Security Requirements
Encryption and Cybersecurity:
Use of advanced encryption standards (AES-256) to secure all communications between satellites and ground control.
Implementation of quantum encryption methods to further enhance security against potential quantum computer attacks.
Continuous monitoring and updating of cybersecurity measures to defend against new and emerging threats.
Access Control:
Multi-factor authentication (MFA) and biometric verification for system access.
Role-based access control (RBAC) to limit the functionality available to different users based on their roles.
Physical Security:
Secure, hardened ground stations with physical barriers and surveillance systems.
Anti-tampering mechanisms in satellite hardware to detect and prevent unauthorized modifications.
Mathematical and Scientific Requirements
Signal Processing and Detection Algorithms:
Development of algorithms for real-time signal processing to detect and identify hostile communications.
Use of machine learning models to improve the accuracy of threat detection and response.
Optics and Laser Physics:
Research into high-energy laser physics and optics to create focused beams capable of non-lethal intervention.
Development of adaptive optics to correct for atmospheric disturbances and ensure precise targeting.
Weather Control and Atmospheric Science:
Advanced models for weather prediction and control, incorporating satellite data to influence weather patterns (as depicted in the images).
Research into the generation and control of phenomena like tornadoes and lightning for defensive purposes.
Engineering Requirements
Satellite Design and Manufacturing:
Use of lightweight, durable materials for satellite construction to withstand space conditions.
Miniaturization of components to fit advanced technology within the satellite's payload capacity.
Incorporation of solar panels and other energy sources for sustained operations.
Laser and Microwave Technology:
High-power, directed-energy systems capable of delivering precise, non-lethal interventions.=non-liable outcome.
Cooling systems and power management to support the continuous operation of these systems.
Telecommunication:
Reliable, redundant communication links between satellites and ground control to ensure continuous operation.
High-bandwidth data transmission systems for real-time control and feedback.
Technologies Required
Advanced Sensors:
High-resolution imaging sensors for surveillance and targeting.
Thermal imaging and x-ray technology for identifying hidden threats.
Artificial Intelligence:
AI algorithms for threat detection, identification, and decision-making.
Machine learning to continuously improve system performance and adapt to new threats.
Advanced materials that can absorb or deflect incoming attacks.
Integration of Images
The images provided depict various scenarios and functionalities of the SRG system. Here's a detailed look at each:
Stealth Satellite Weather Controlling:
Illustrates the use of satellites to influence weather patterns for defense.
Requires advanced atmospheric models and control algorithms.
Psychological Operations:
Depicts the use of psychological tactics to deter hostile actions.
Involves AI for voice and behavior analysis.
Energy and Environmental Control:
Shows the control of environmental elements like lightning and tornadoes.
Requires real-time atmospheric data and control mechanisms.
Anti-ICBM and Advanced Missile Defense:
Depicts the use of high-energy lasers and other systems for missile defense.
Involves precise targeting systems and high-power energy sources.
General Surveillance and Operations:
Highlights the surveillance capabilities of the SRG system.
Requires advanced imaging and signal processing technologies.
Conclusion
The SRG system by Nick Folkes envisions a highly advanced, multi-functional satellite-based defense system. Implementing this system would require cutting-edge technologies in various fields, robust security measures, and continuous advancements in AI and machine learning. The integration of these elements would create a formidable defense mechanism capable of deterring and neutralizing hostile actions effectively.
Further analysis and refinement of the concepts based on the remaining images will add more specific details to this comprehensive overview, ensuring that every aspect of the SRG system is addressed and optimized.
3)
To develop the SRG (Satellite Ray Gun) system as proposed by Nick Folkes, we need to focus on several advanced fields of science and engineering. Here is an in-depth analysis:
Security
1. Encryption and Cybersecurity:
Advanced Encryption Standard (AES-256): Widely adopted encryption standard to secure communications.
Quantum Encryption: Implementing quantum key distribution (QKD) ensures security against future quantum computer threats.
Intrusion Detection Systems (IDS): For continuous monitoring of network traffic and alerting on potential breaches.
2. Access Control:
Biometric Authentication: Fingerprint, retina scans, and facial recognition to ensure only authorized personnel access the systems.
Role-Based Access Control (RBAC): Restricting access based on user roles to minimize the risk of internal threats.
3. Physical Security:
Hardened Facilities: Using reinforced structures and secure locations for ground control stations.
Anti-Tampering Measures: Equipping satellites with sensors that detect physical tampering or unauthorized access.
Mathematical and Scientific Foundations
1. Signal Processing and Detection Algorithms:
Fourier Transforms: For analyzing frequencies within signals.
Kalman Filters: To predict and filter out noise in data streams.
Machine Learning Models: Neural networks and deep learning for pattern recognition and anomaly detection.
Equations:
Fourier Transform: F(k)=∫−∞∞f(x)e−2πikxdxF(k)=∫−∞∞f(x)e−2πikxdx
Kalman Filter:x^k∣k=x^k∣k−1+Kk(zk−Hx^k∣k−1)Kk=Pk∣k−1HT(HPk∣k−1HT+R)−1Pk∣k=(I−KkH)Pk∣k−1x^k∣kKkPk∣k=x^k∣k−1+Kk(zk−Hx^k∣k−1)=Pk∣k−1HT(HPk∣k−1HT+R)−1=(I−KkH)Pk∣k−1
2. Optics and Laser Physics:
Beam Focusing: Using adaptive optics to correct atmospheric distortions.
Laser Cooling: Techniques to maintain the operational stability of high-energy lasers.
Equations:
Gaussian Beam Optics:I(r,z)=I0(w0w(z))2exp(−2r2w(z)2)I(r,z)=I0(w(z)w0)2exp(−2w(z)2r2) where w(z)=w01+(zλπw02)2w(z)=w01+(πw02zλ)2.
3. Weather Control and Atmospheric Science:
Numerical Weather Prediction (NWP): Using mathematical models to predict weather changes.
Atmospheric Ionization: Modifying atmospheric ions to influence weather patterns.
Equations:
Navier-Stokes Equation for fluid dynamics in weather systems:∂u∂t+(u⋅∇)u=−∇pρ+ν∇2u+f∂t∂u+(u⋅∇)u=−ρ∇p+ν∇2u+f
Engineering Requirements
1. Satellite Design and Manufacturing:
Composite Materials: Lightweight and strong materials such as carbon fiber composites.
Microelectromechanical Systems (MEMS): For miniaturization of sensors and actuators.
2. Laser and Microwave Technology:
Solid-State Lasers: High-power lasers with solid gain media.
Gyrotrons: High-frequency microwave generators for directed energy weapons.
3. Telecommunication:
High-Bandwidth Communication Links: Utilizing microwave and laser communication for fast data transfer.
Redundant Systems: Ensuring continuous operation even if some components fail.
Technologies Required
1. Advanced Sensors:
LIDAR: Light detection and ranging for high-resolution mapping.
Hyperspectral Imaging: Capturing a wide spectrum of light to identify materials.
2. Artificial Intelligence:
Deep Learning: Convolutional neural networks (CNN) for image and signal analysis.
Reinforcement Learning: For autonomous decision-making in threat response.
3. Robotics and Automation:
Autonomous Drones: For in-orbit repairs and adjustments.
Robotic Arms: For precision tasks in space.
Forcefields and Defensive Measures
1. Electromagnetic Shields:
Plasma Windows: Creating plasma fields to act as barriers.
Magnetohydrodynamic (MHD) Shields: Using magnetic fields to deflect charged particles.
Equations:
Maxwell's Equations for electromagnetism:∇⋅E=ρϵ0,∇⋅B=0∇×E=−∂B∂t,∇×B=μ0J+μ0ϵ0∂E∂t∇⋅E∇×E=ϵ0ρ,∇⋅B=0=−∂t∂B,∇×B=μ0J+μ0ϵ0∂t∂E
2. Kinetic Barriers:
Deployable Barriers: Using advanced materials like graphene composites for kinetic protection.
Development Timeline
Phase 1: Research and Development (5-7 years)
Conduct foundational research in advanced materials, quantum encryption, and AI algorithms.
Develop prototype systems for testing.
Phase 2: Testing and Iteration (3-5 years)
Field test prototypes and refine based on results.
Develop and test forcefield technologies and defensive measures.
Phase 3: Deployment (3-5 years)
Full-scale production of SRG satellites and ground systems.
Gradual deployment and integration into defense systems.
Total Estimated Time: 11-17 years
Conclusion
The development of the SRG system requires cutting-edge advancements in multiple fields. Implementing robust security measures, advanced mathematical models, and engineering innovative satellite systems will be essential. While ambitious, the realization of Nick Folkes' designs will propel defense technology into a new era, with forcefields and autonomous systems forming the backbone of future security infrastructure.
To make the SRG (Satellite Ray Gun) system successful in deterring hostile aggressions, a combination of advanced security, mathematical, scientific, and engineering principles and technologies is required. Below is an analysis based on the provided descriptions and images of the SRG system:
Security Requirements
Encryption and Cybersecurity:
Advanced encryption algorithms to secure communications between satellites and ground stations.
Robust cybersecurity measures to protect the system from hacking and cyber-attacks.
Regular security audits and updates to address potential vulnerabilities.
Access Control:
Multi-factor authentication for access to the system’s control interfaces.
Strict access control policies to ensure only authorized personnel can operate or modify the system.
Physical Security:
Secure locations for ground control stations.
Anti-tamper mechanisms on satellite hardware.
Mathematical and Scientific Requirements
Signal Processing:
Algorithms for detecting and identifying hostile communications and actions.
Real-time data processing to enable immediate response to threats.
Physics and Optics:
Understanding of laser and microwave physics for the development of the ray gun technology.
Advanced optics for targeting and focusing the ray beams accurately.
Astrodynamics:
Orbital mechanics to position and maintain satellites in optimal locations.
Calculations for satellite trajectories and targeting mechanisms.
Engineering Requirements
Satellite Design and Manufacturing:
Durable materials and components to withstand the harsh conditions of space.
Miniaturization of components to fit advanced technology within the satellite’s payload capacity.
Laser and Microwave Technology:
Development of high-power, non-lethal laser and microwave systems.
Cooling and power systems to support continuous operation.
Integration of Images
To fully integrate the information provided in the images into this analysis, each image will be examined for its specific technological and operational details. Here is a brief overview of the images and their potential contributions:
SRG Without and With Speech Jammer Gun:
Highlights the effectiveness of the speech jammer in silencing hostile communications.
Space Battle and Defense Mechanisms:
Depicts the satellite and ground-based defense systems in action.
Anti-Abuse Tower:
Illustrates the concept of a remote tower equipped with ray guns for deterring hostiles.
Technical Descriptions:
Detailed descriptions of the various features and capabilities of the SRG system.
Operational Scenarios:
Examples of how the SRG system can be deployed in different scenarios to counter threats.
Conclusion
The SRG system by Nick Folkes requires a multifaceted approach involving state-of-the-art technology, advanced scientific principles, and robust security measures. Implementing this system would involve extensive research and development in various fields, from satellite engineering to advanced optics and AI. Additionally, forcefields and defensive measures would need to be integrated to ensure the system's resilience against external threats.
Once the remaining images are analyzed, further details can be added to this overview to create a comprehensive plan for the SRG system’s implementation.
--------------------------------------------------------------------
(part. ii/iii batch)
Pictures 10-17:
Secure, hardened ground stations with physical barriers and surveillance systems.
Use of lightweight, durable materials for satellite construction to withstand space conditions.
Miniaturization of components to fit advanced technology within the satellite's payload capacity.
Incorporation of solar panels and other energy sources for sustained operations.
Laser and Microwave Technology:
High-power, directed-energy systems capable of delivering precise, non-lethal interventions.
Cooling systems and power management to support the continuous operation of these systems.
Telecommunication:
Reliable, redundant communication links between satellites and ground control to ensure continuous operation.
High-bandwidth data transmission systems for real-time control and feedback.
Technologies Required
Advanced Sensors:
High-resolution imaging sensors for surveillance and targeting.
Thermal imaging and x-ray technology for identifying hidden threats.
Artificial Intelligence:
AI algorithms for threat detection, identification, and decision-making.
Machine learning to continuously improve system performance and adapt to new threats.
Robotics and Automation:
Automated systems for satellite maintenance and operation.
Robotic arms for in-orbit repairs and adjustments.
Forcefields and Defensive Measures
Electromagnetic Shields:
Development of electromagnetic field generators to create protective shields around satellites.
Research into materials and technologies that can generate and sustain these fields effectively.
Kinetic Barriers:
Deployable kinetic barriers to protect against physical projectiles.
Advanced materials that can absorb or deflect incoming attacks.
General Surveillance and Operations:
Highlights the surveillance capabilities of the SRG system.
Requires advanced imaging and signal processing technologies.
Conclusion
The SRG system by Nick Folkes envisions a highly advanced, multi-functional satellite-based defense system. Implementing this system would require cutting-edge technologies in various fields, robust security measures, and continuous advancements in AI and machine learning. The integration of these elements would create a formidable defense mechanism capable of deterring and neutralizing hostile actions effectively.
Further analysis and refinement of the concepts based on the remaining images will add more specific details to this comprehensive overview, ensuring that every aspect of the SRG system is addressed and optimized.
----------------------------------------------------------
(part. iii/iii batch)
To develop the SRG (Satellite Ray Gun) system as proposed by Nick Folkes, we need to focus on several advanced fields of science and engineering. Here is an in-depth analysis:
Security
1. Encryption and Cybersecurity:
Advanced Encryption Standard (AES-256): Widely adopted encryption standard to secure communications.
Quantum Encryption: Implementing quantum key distribution (QKD) ensures security against future quantum computer threats.
Intrusion Detection Systems (IDS): For continuous monitoring of network traffic and alerting on potential breaches.
2. Access Control:
Biometric Authentication: Fingerprint, retina scans, and facial recognition to ensure only authorized personnel access the systems.
Role-Based Access Control (RBAC): Restricting access based on user roles to minimize the risk of internal threats.
3. Physical Security:
Hardened Facilities: Using reinforced structures and secure locations for ground control stations.
Anti-Tampering Measures: Equipping satellites with sensors that detect physical tampering or unauthorized access.
Mathematical and Scientific Foundations
1. Signal Processing and Detection Algorithms:
Fourier Transforms: For analyzing frequencies within signals.
Kalman Filters: To predict and filter out noise in data streams.
Machine Learning Models: Neural networks and deep learning for pattern recognition and anomaly detection.
Equations:
Fourier Transform: F(k)=∫−∞∞f(x)e−2πikxdxF(k)=∫−∞∞f(x)e−2πikxdx
Kalman Filter:x^k∣k=x^k∣k−1+Kk(zk−Hx^k∣k−1)Kk=Pk∣k−1HT(HPk∣k−1HT+R)−1Pk∣k=(I−KkH)Pk∣k−1x^k∣kKkPk∣k=x^k∣k−1+Kk(zk−Hx^k∣k−1)=Pk∣k−1HT(HPk∣k−1HT+R)−1=(I−KkH)Pk∣k−1
2. Optics and Laser Physics:
Beam Focusing: Using adaptive optics to correct atmospheric distortions.
Laser Cooling: Techniques to maintain the operational stability of high-energy lasers.
Equations:
Gaussian Beam Optics:I(r,z)=I0(w0w(z))2exp(−2r2w(z)2)I(r,z)=I0(w(z)w0)2exp(−2w(z)2r2) where w(z)=w01+(zλπw02)2w(z)=w01+(πw02zλ)2.
3. Weather Control and Atmospheric Science:
Numerical Weather Prediction (NWP): Using mathematical models to predict weather changes.
Atmospheric Ionization: Modifying atmospheric ions to influence weather patterns.
Equations:
Navier-Stokes Equation for fluid dynamics in weather systems:∂u∂t+(u⋅∇)u=−∇pρ+ν∇2u+f∂t∂u+(u⋅∇)u=−ρ∇p+ν∇2u+f
Engineering Requirements
1. Satellite Design and Manufacturing:
Composite Materials: Lightweight and strong materials such as carbon fiber composites.
Microelectromechanical Systems (MEMS): For miniaturization of sensors and actuators.
2. Laser and Microwave Technology:
Solid-State Lasers: High-power lasers with solid gain media.
Gyrotrons: High-frequency microwave generators for directed energy weapons.
3. Telecommunication:
High-Bandwidth Communication Links: Utilizing microwave and laser communication for fast data transfer.
Redundant Systems: Ensuring continuous operation even if some components fail.
Technologies Required
1. Advanced Sensors:
LIDAR: Light detection and ranging for high-resolution mapping.
Hyperspectral Imaging: Capturing a wide spectrum of light to identify materials.
2. Artificial Intelligence:
Deep Learning: Convolutional neural networks (CNN) for image and signal analysis.
Reinforcement Learning: For autonomous decision-making in threat response.
3. Robotics and Automation:
Autonomous Drones: For in-orbit repairs and adjustments.
Robotic Arms: For precision tasks in space.
Forcefields and Defensive Measures
1. Electromagnetic Shields:
Plasma Windows: Creating plasma fields to act as barriers.
Magnetohydrodynamic (MHD) Shields: Using magnetic fields to deflect charged particles.
Equations:
Maxwell's Equations for electromagnetism:∇⋅E=ρϵ0,∇⋅B=0∇×E=−∂B∂t,∇×B=μ0J+μ0ϵ0∂E∂t∇⋅E∇×E=ϵ0ρ,∇⋅B=0=−∂t∂B,∇×B=μ0J+μ0ϵ0∂t∂E
2. Kinetic Barriers:
Deployable Barriers: Using advanced materials like graphene composites for kinetic protection.
Development Timeline
Phase 1: Research and Development (5-7 years)
Conduct foundational research in advanced materials, quantum encryption, and AI algorithms.
Develop prototype systems for testing.
Phase 2: Testing and Iteration (3-5 years)
Field test prototypes and refine based on results.
Develop and test forcefield technologies and defensive measures.
Phase 3: Deployment (3-5 years)
Full-scale production of SRG satellites and ground systems.
Gradual deployment and integration into defense systems.
Total Estimated Time: 11-17 years
Conclusion
The development of the SRG system requires cutting-edge advancements in multiple fields. Implementing robust security measures, advanced mathematical models, and engineering innovative satellite systems will be essential. While ambitious, the realization of Nick Folkes' designs will propel defense technology into a new era, with forcefields and autonomous systems forming the backbone of future security infrastructure.
=======================================
#(2):
"Base ((Space-Force)-Field)) Iron Dome":
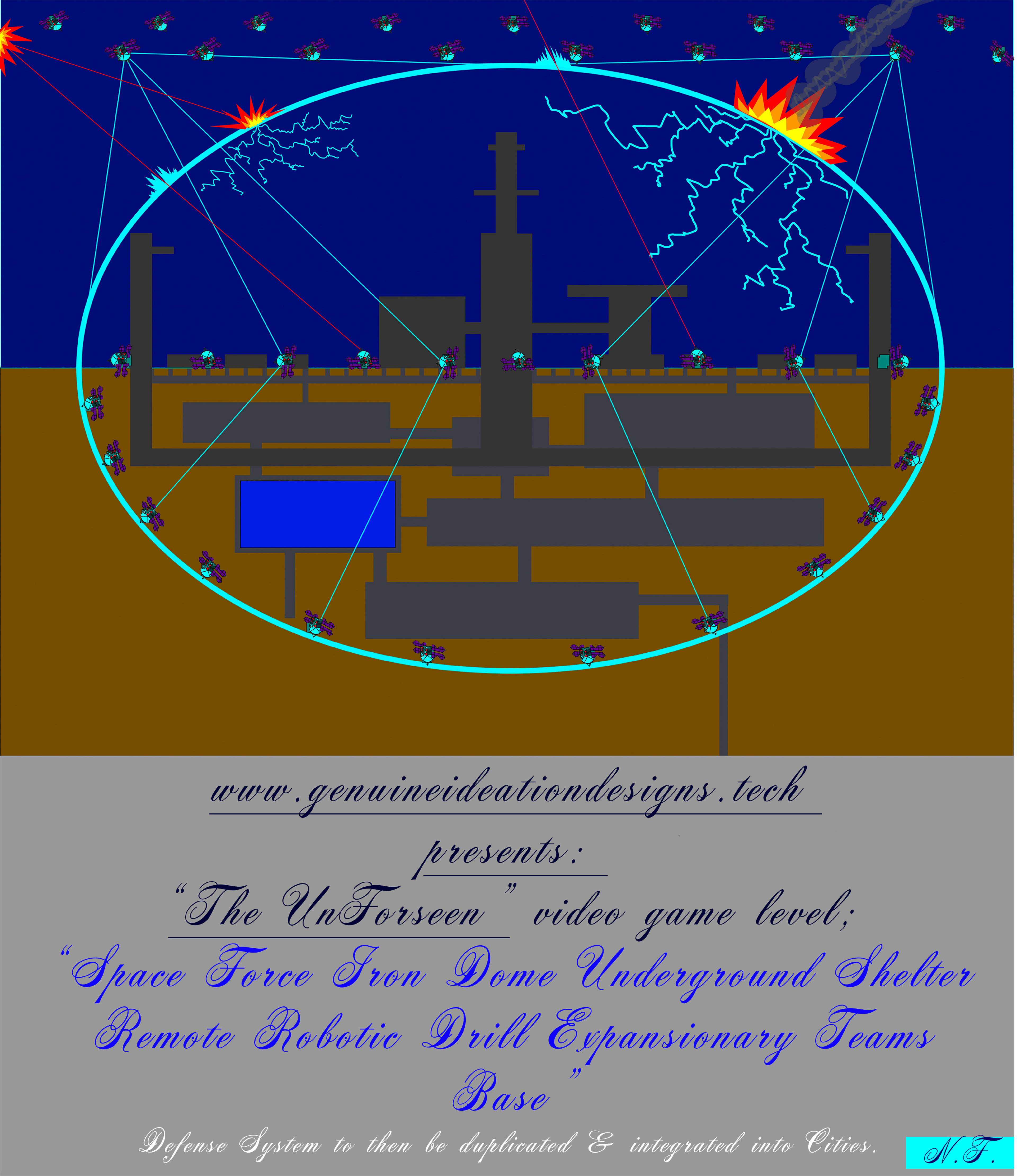
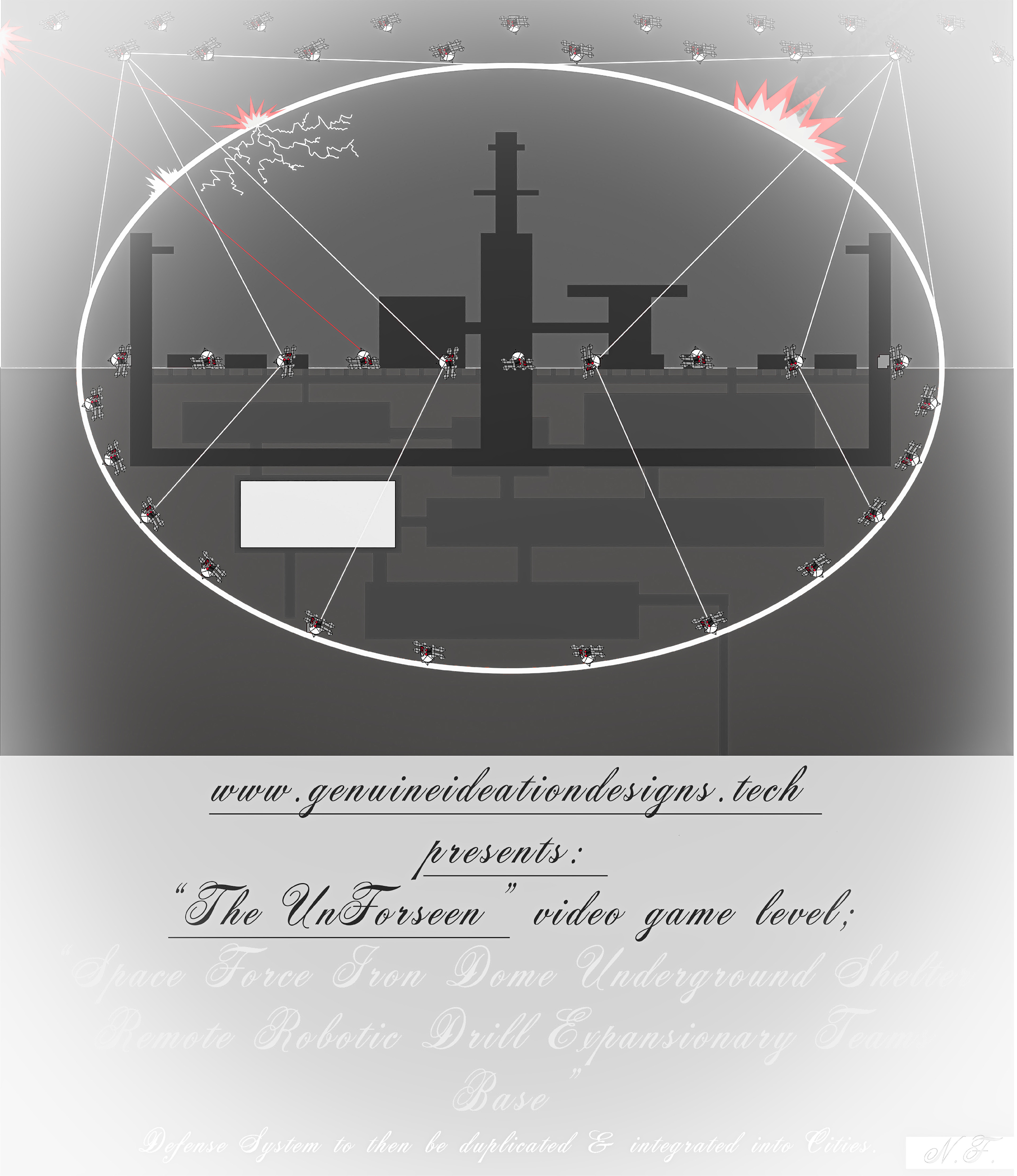
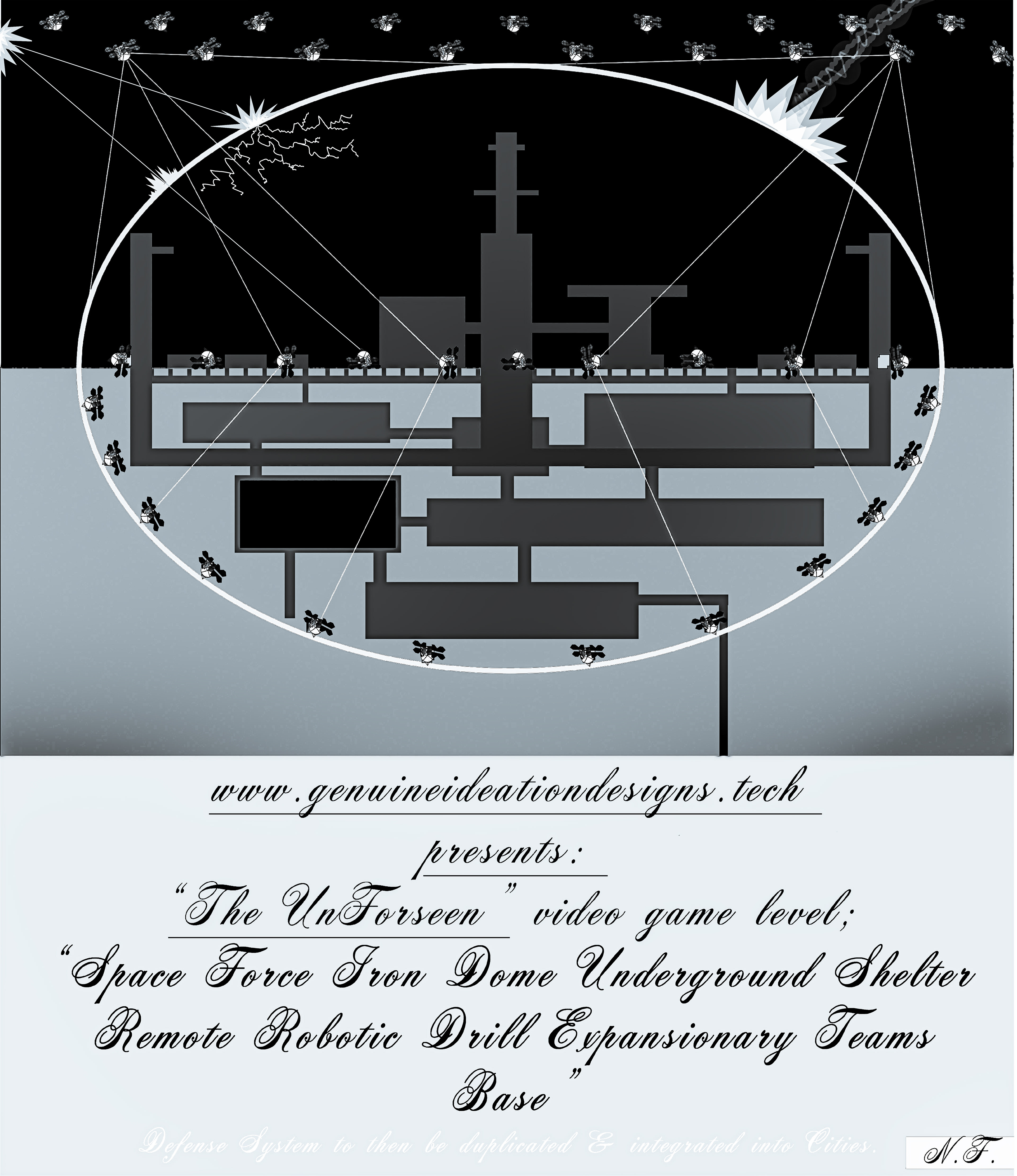
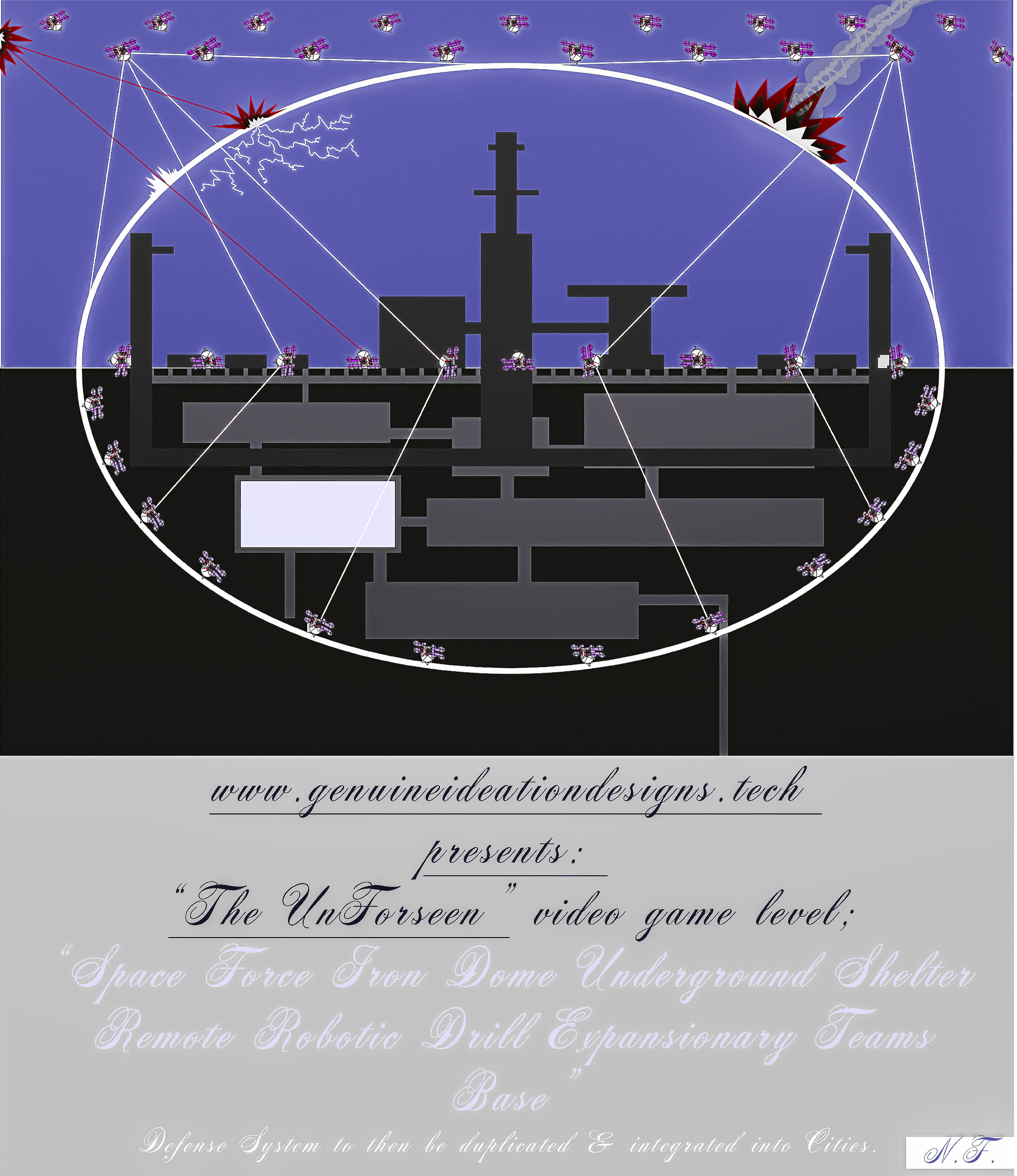
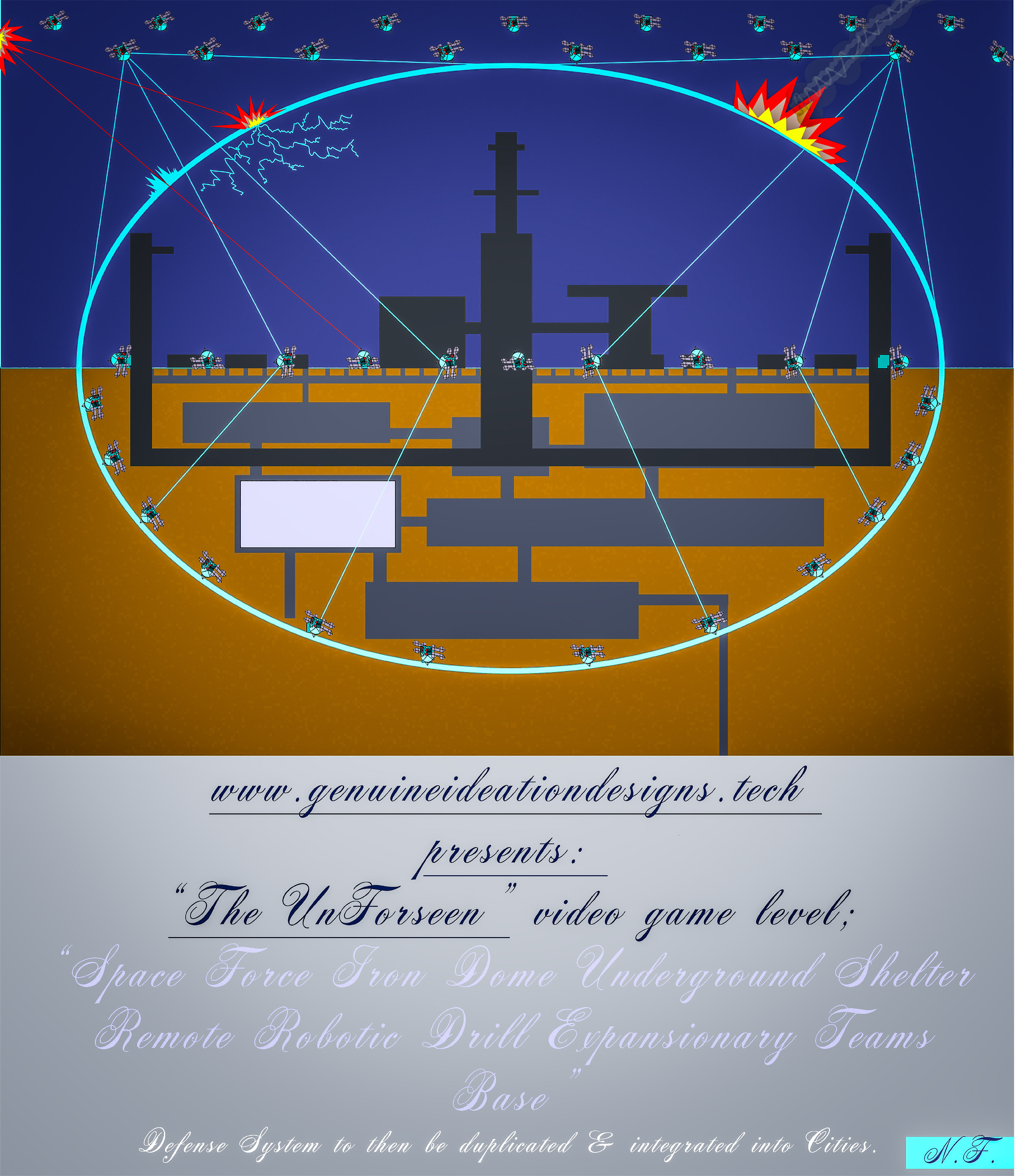
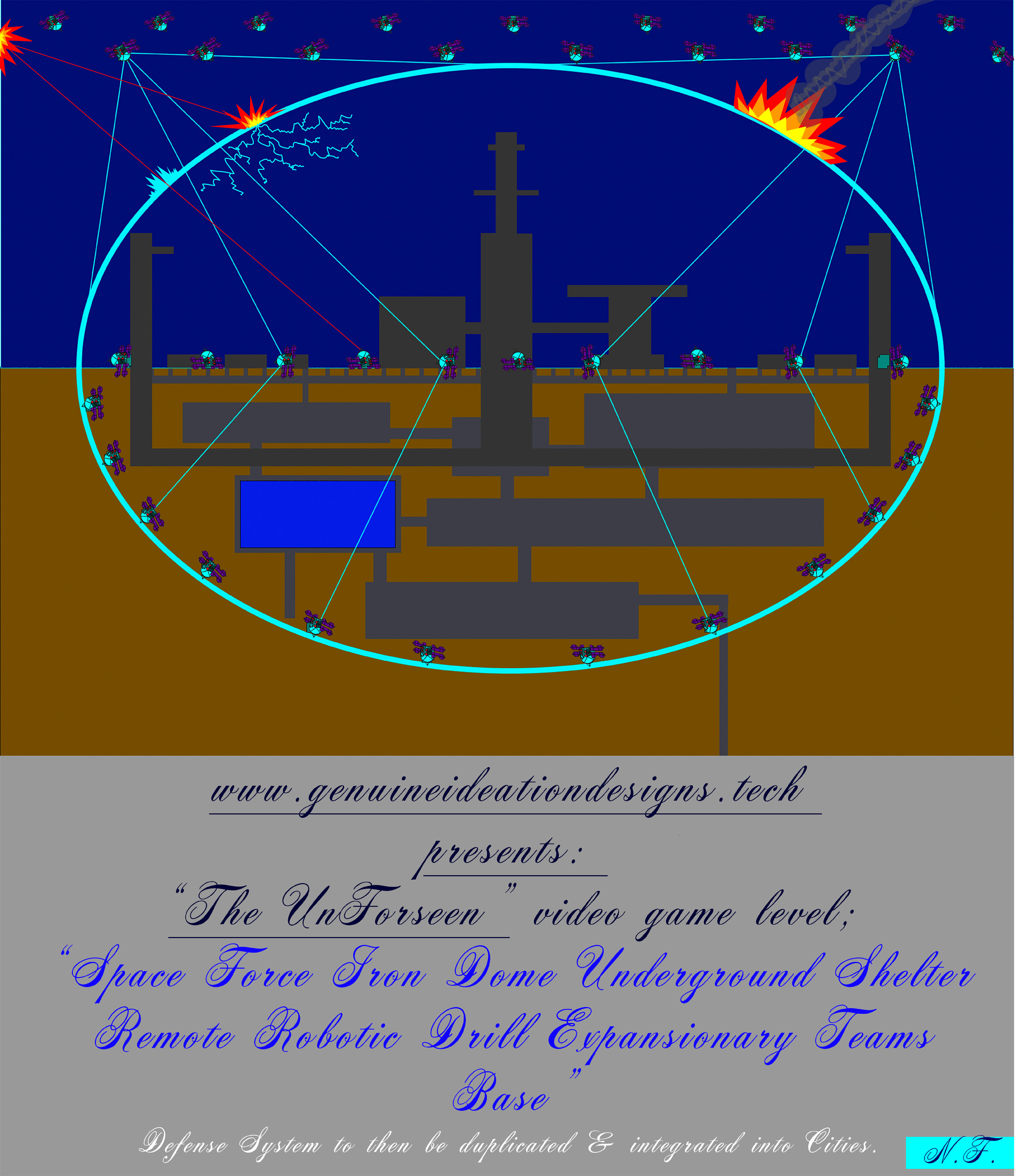
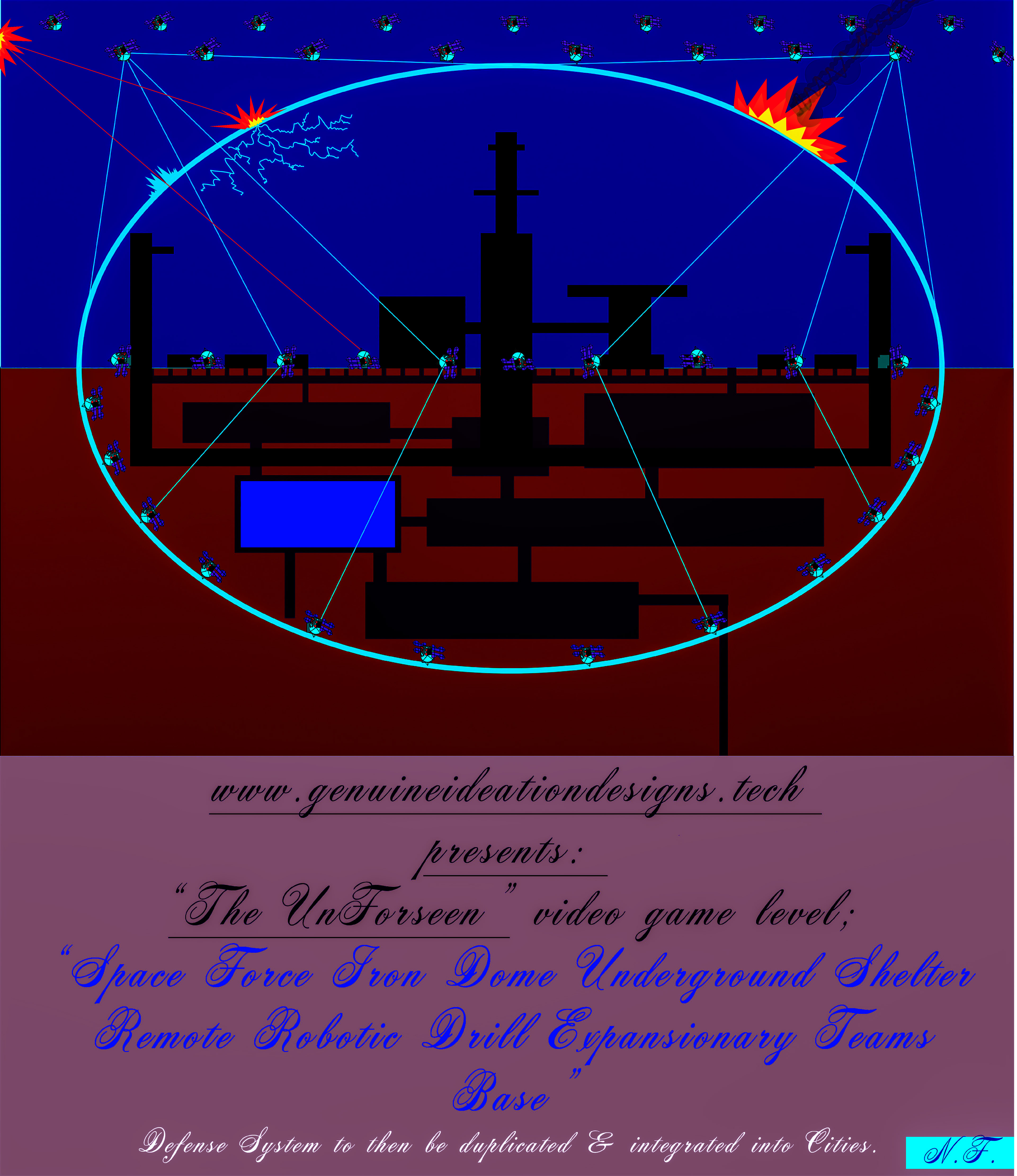
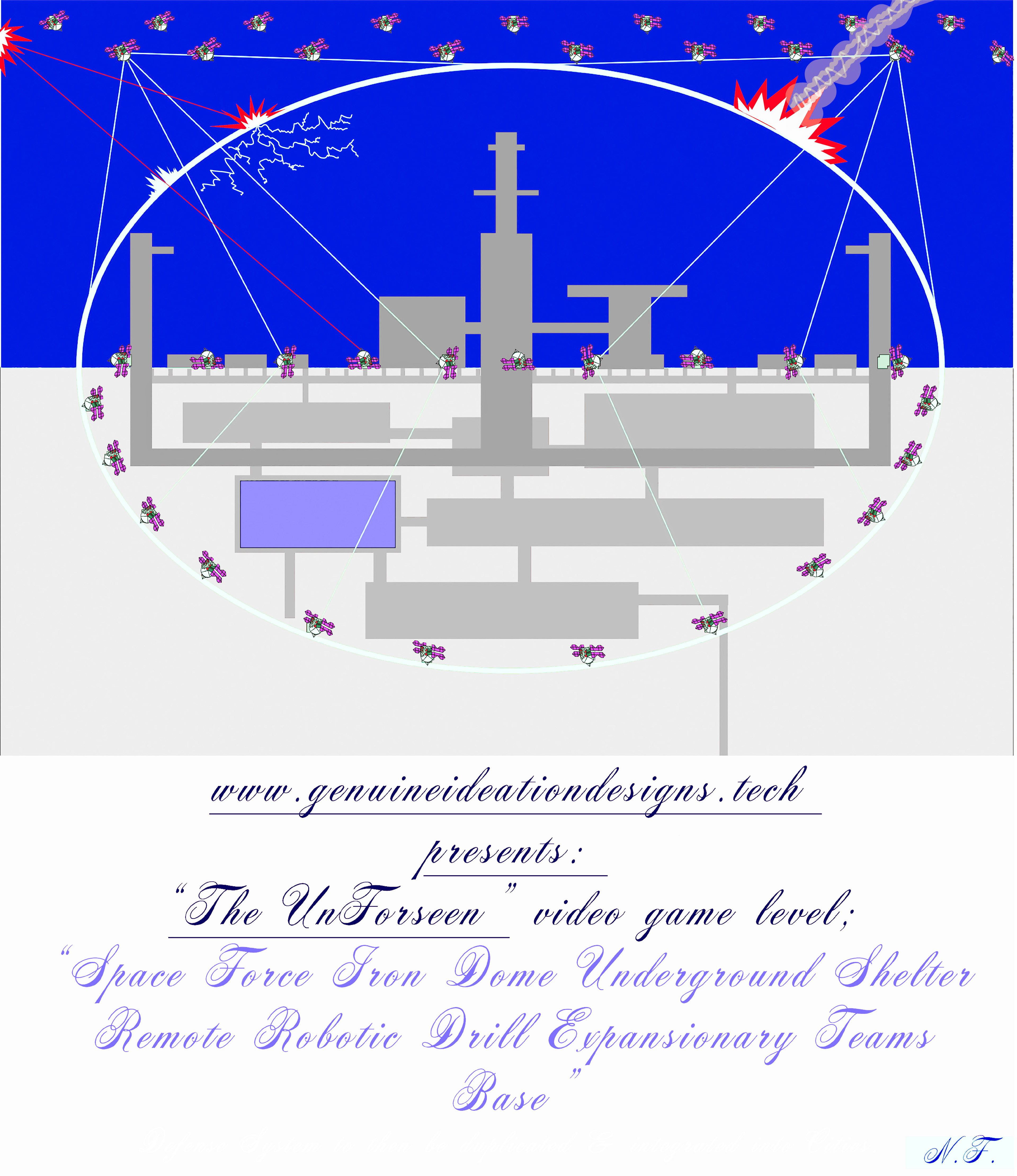
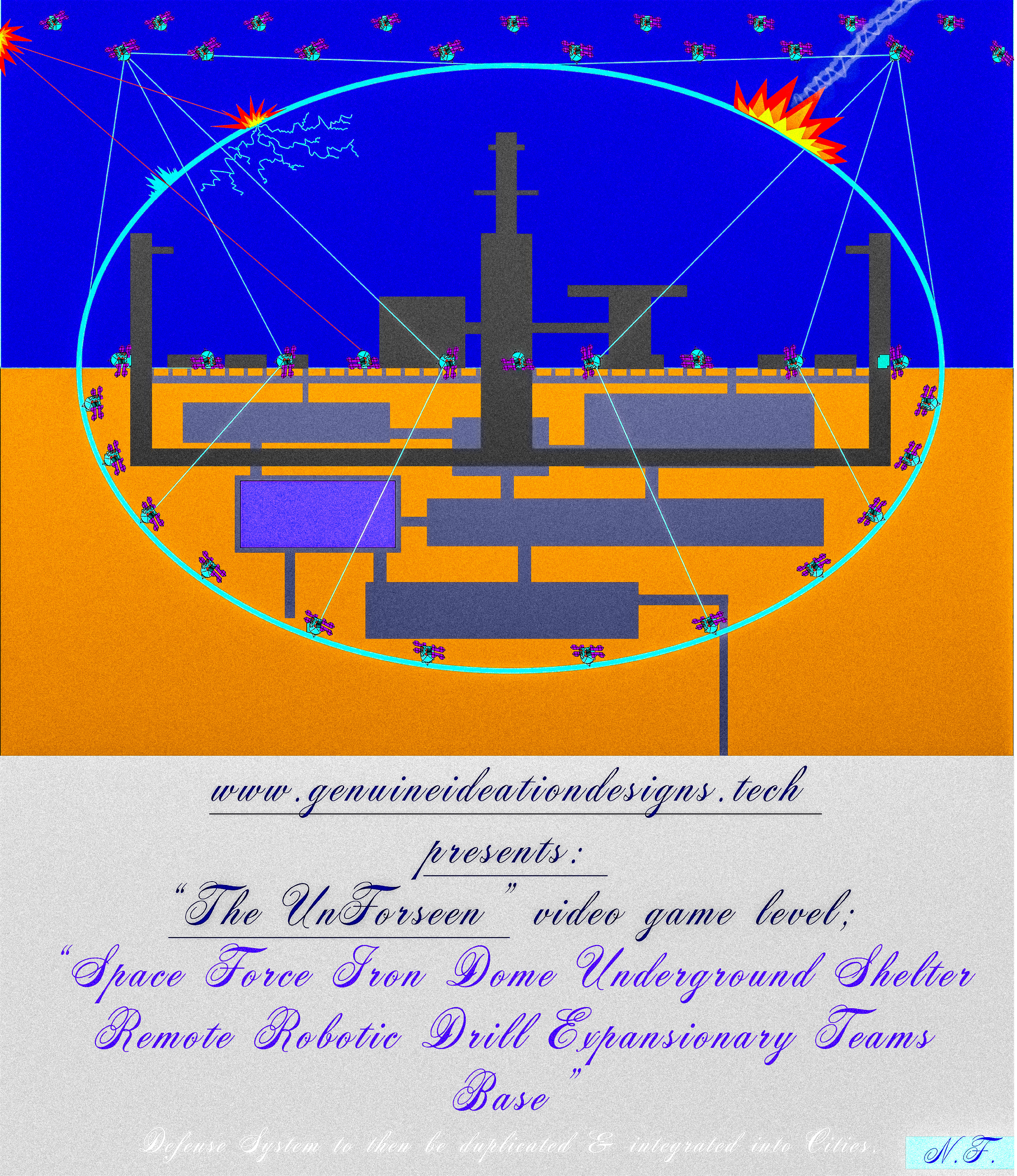
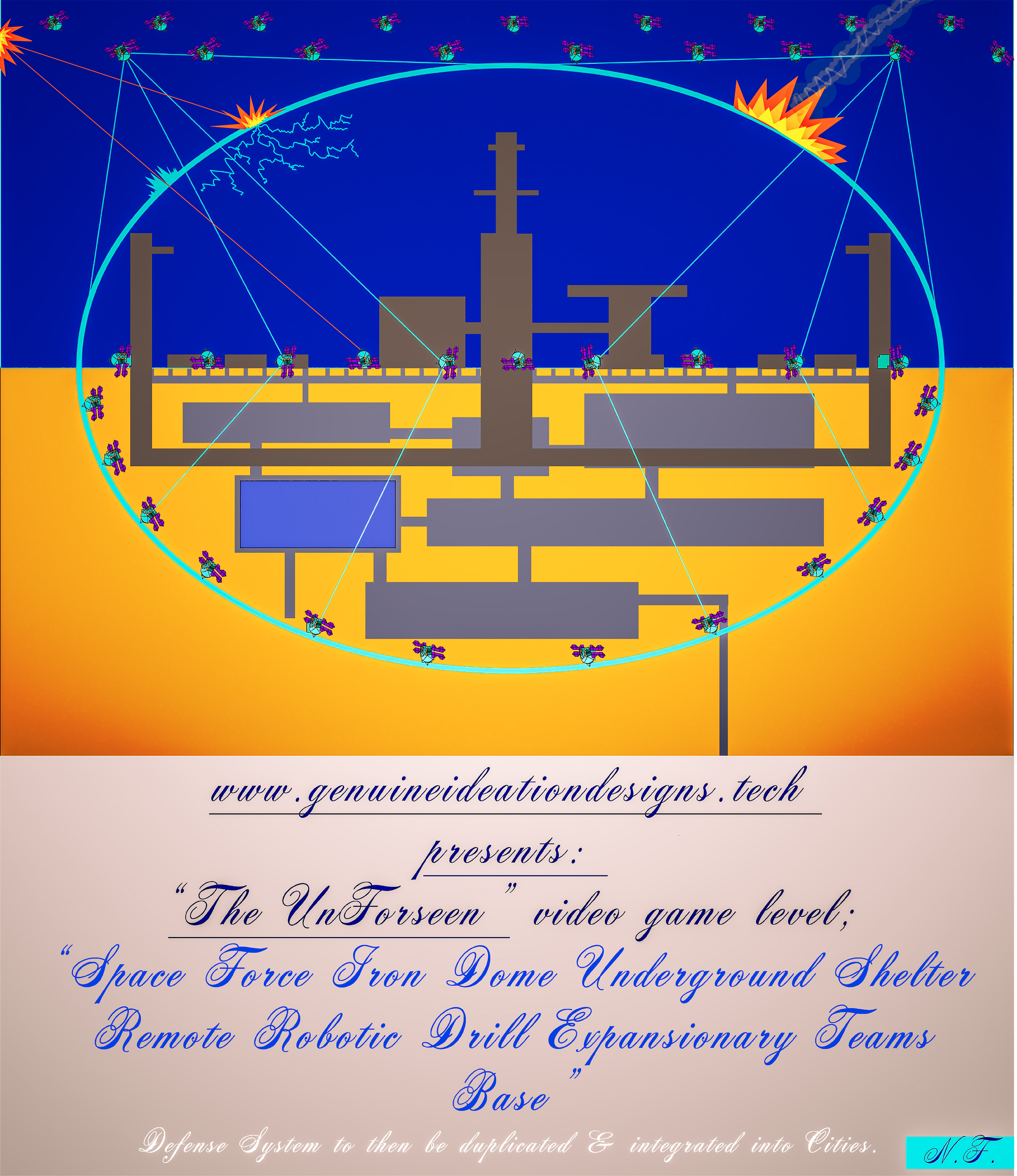
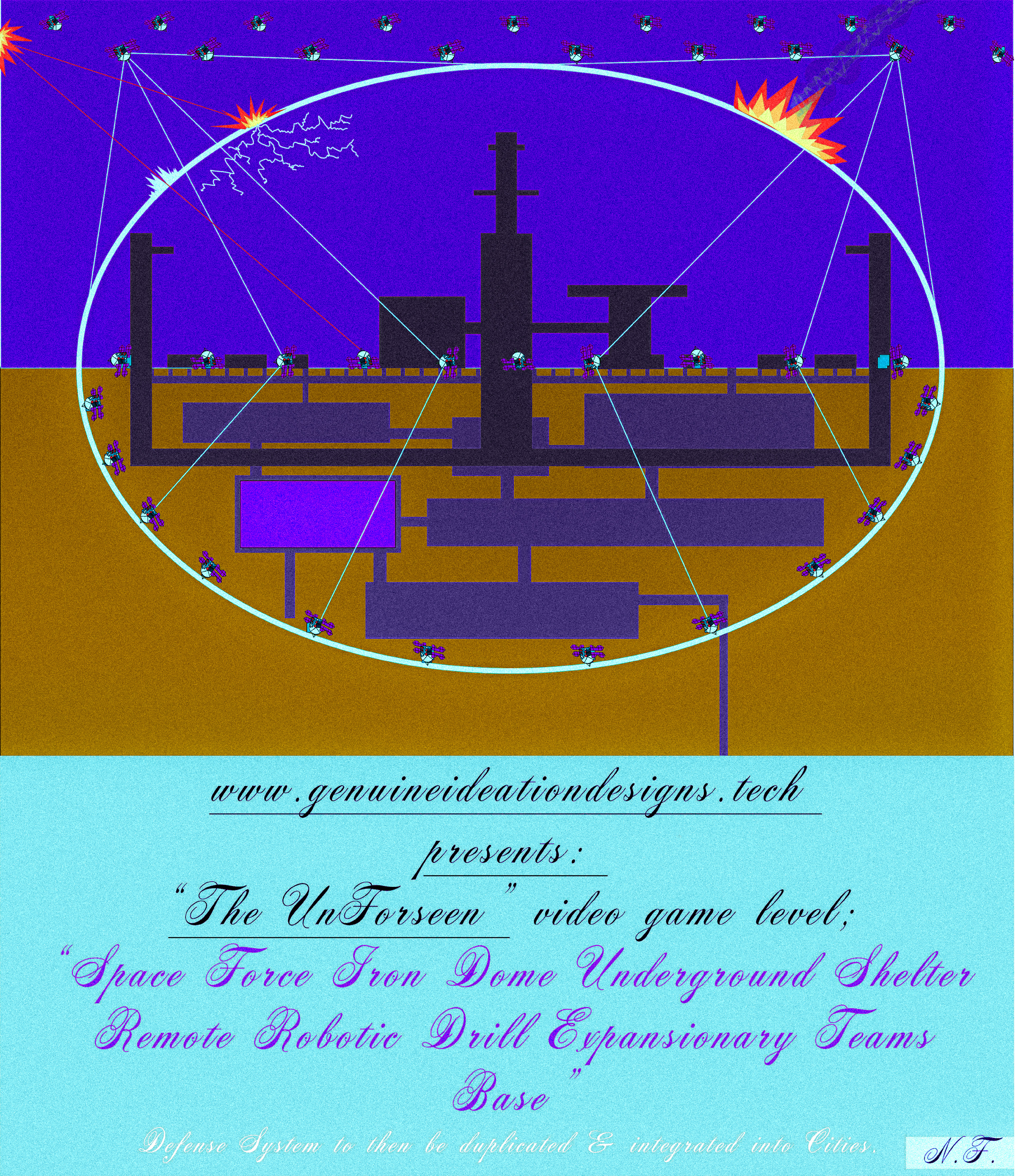
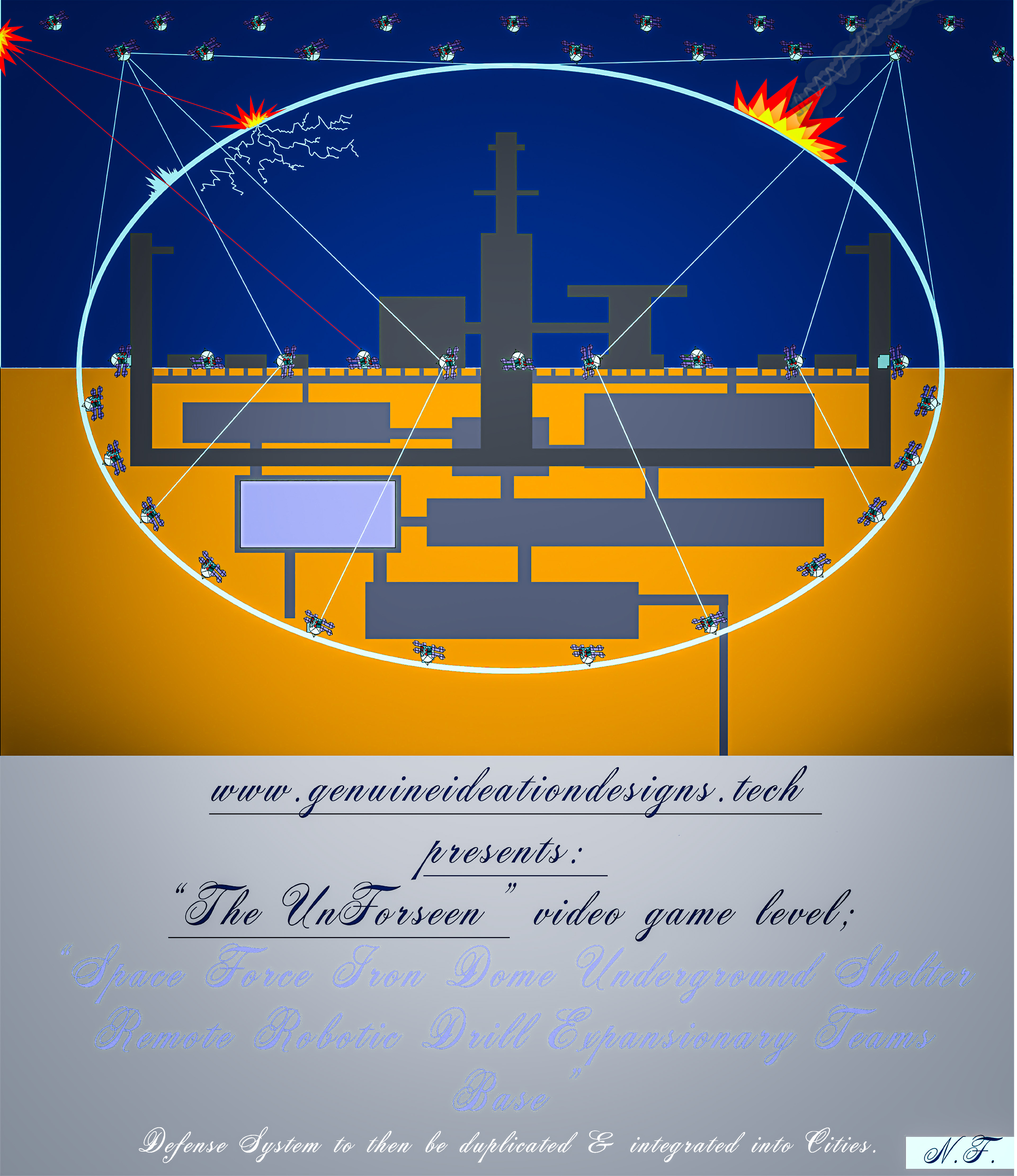
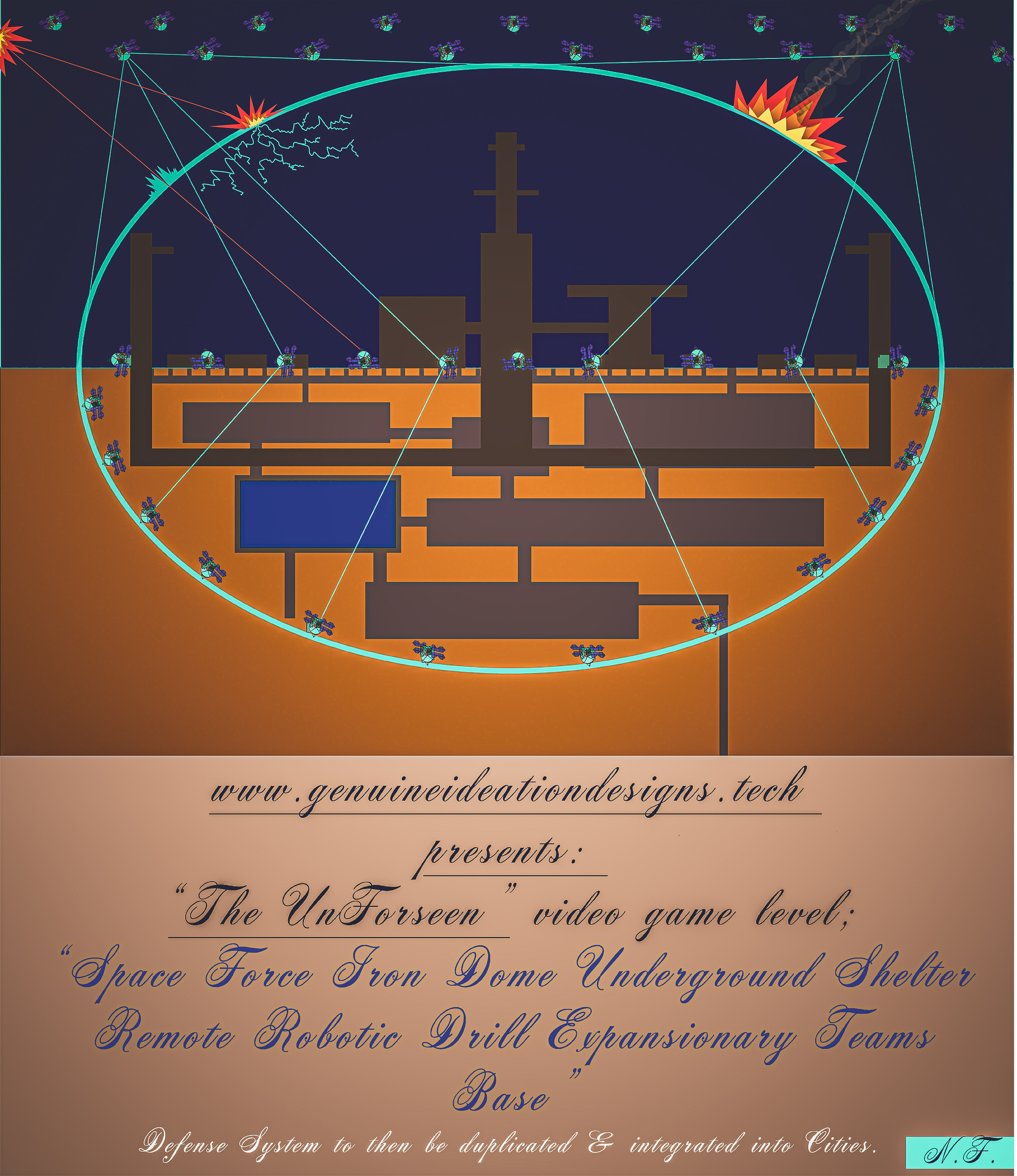
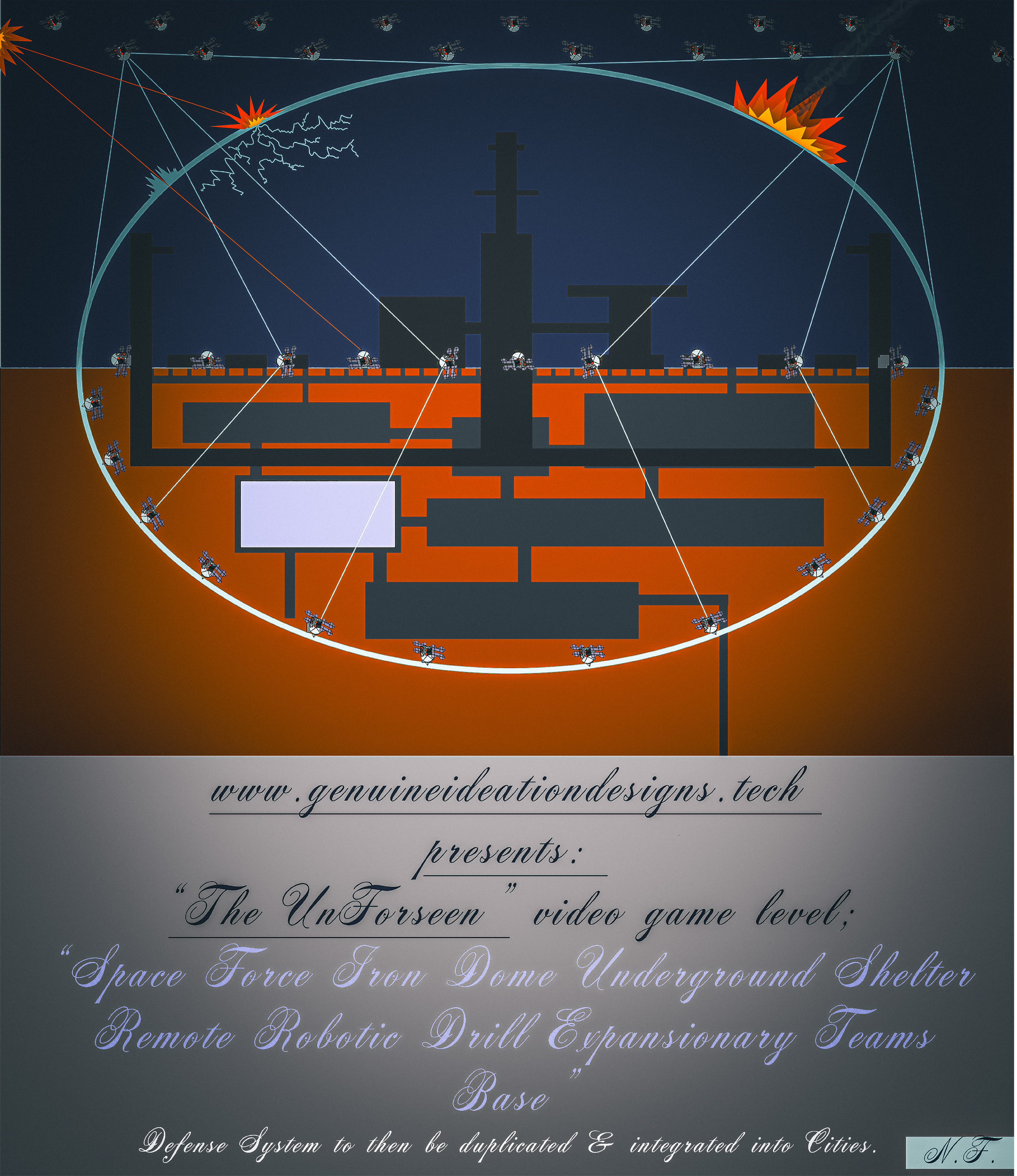
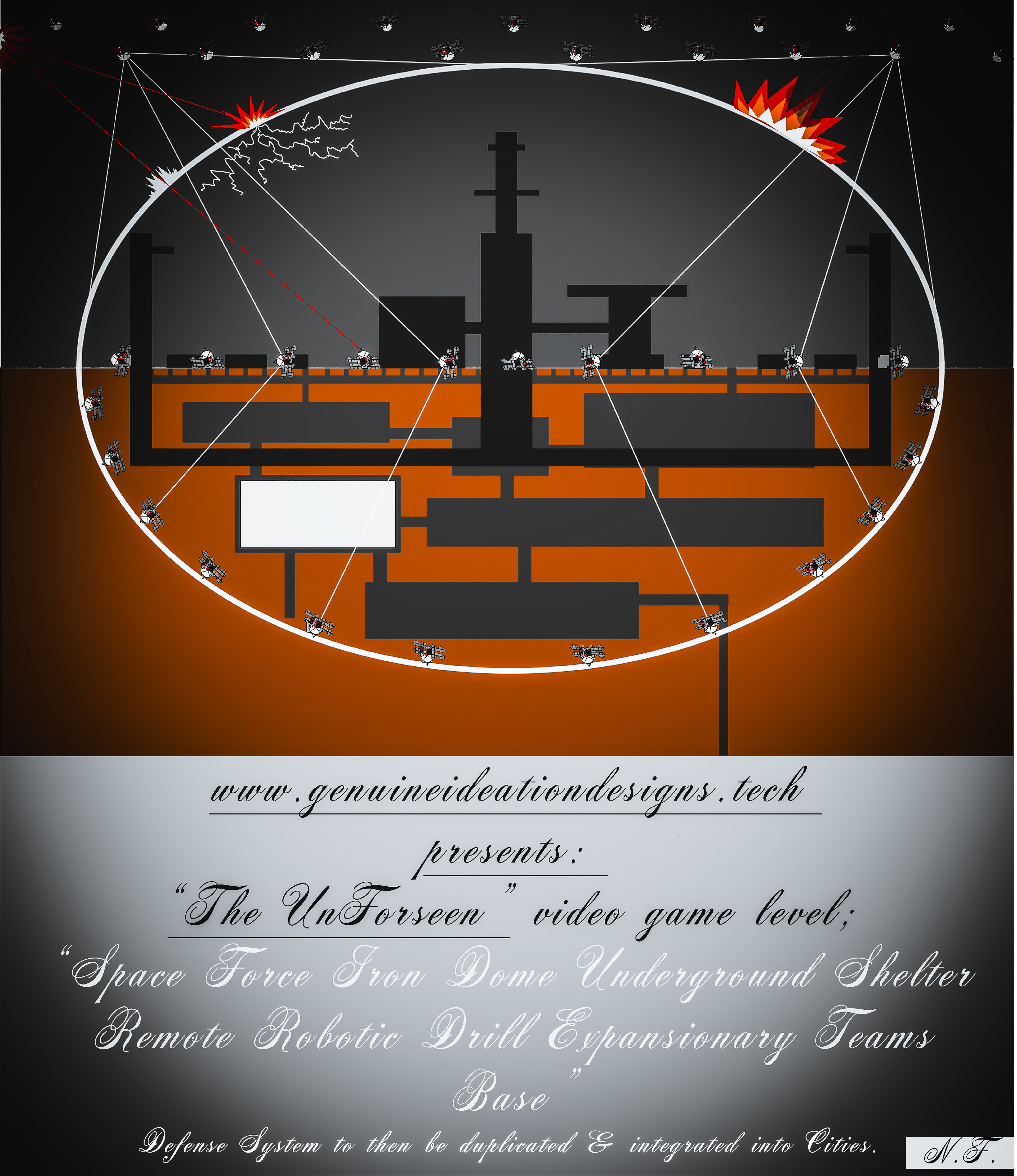
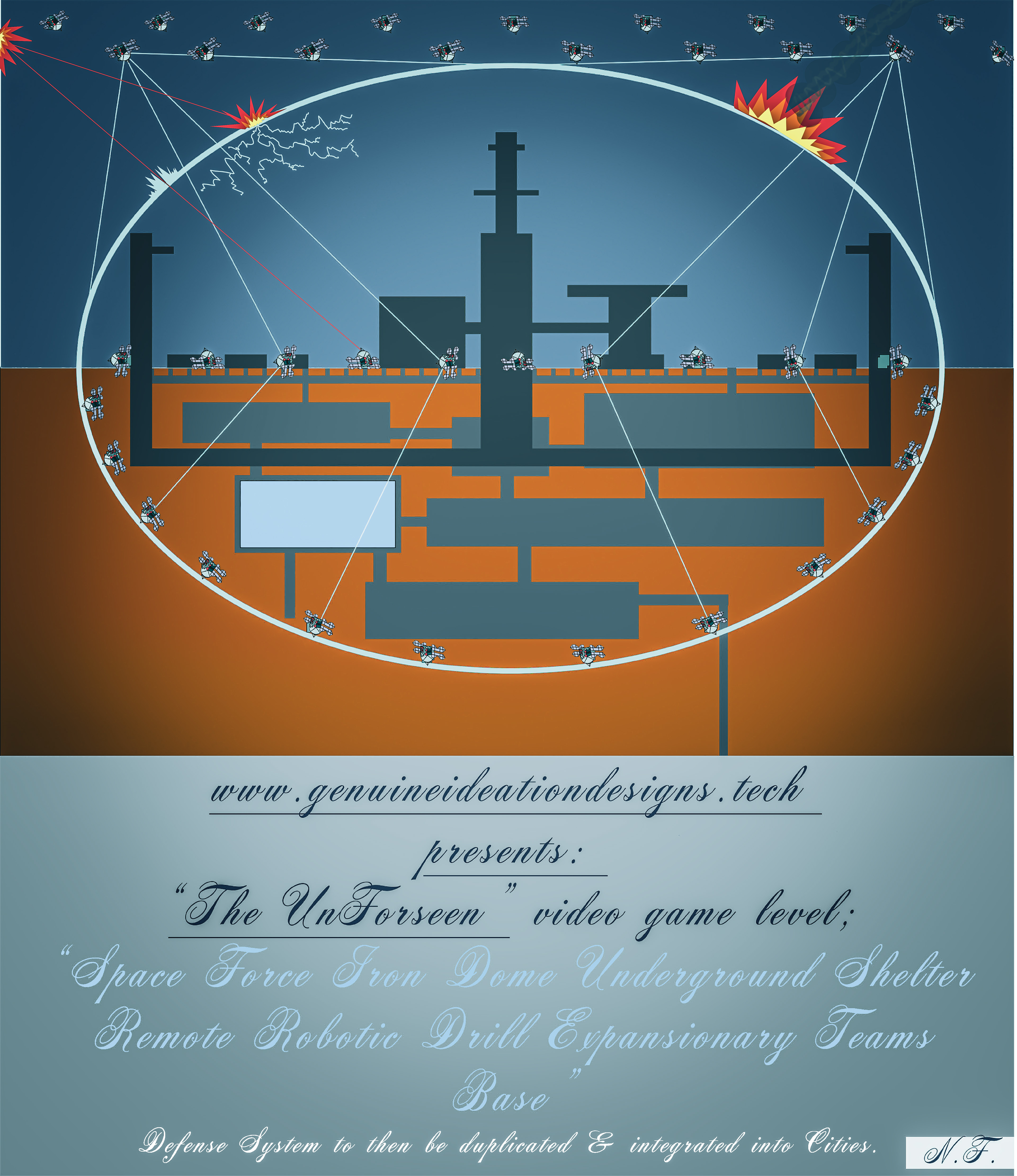
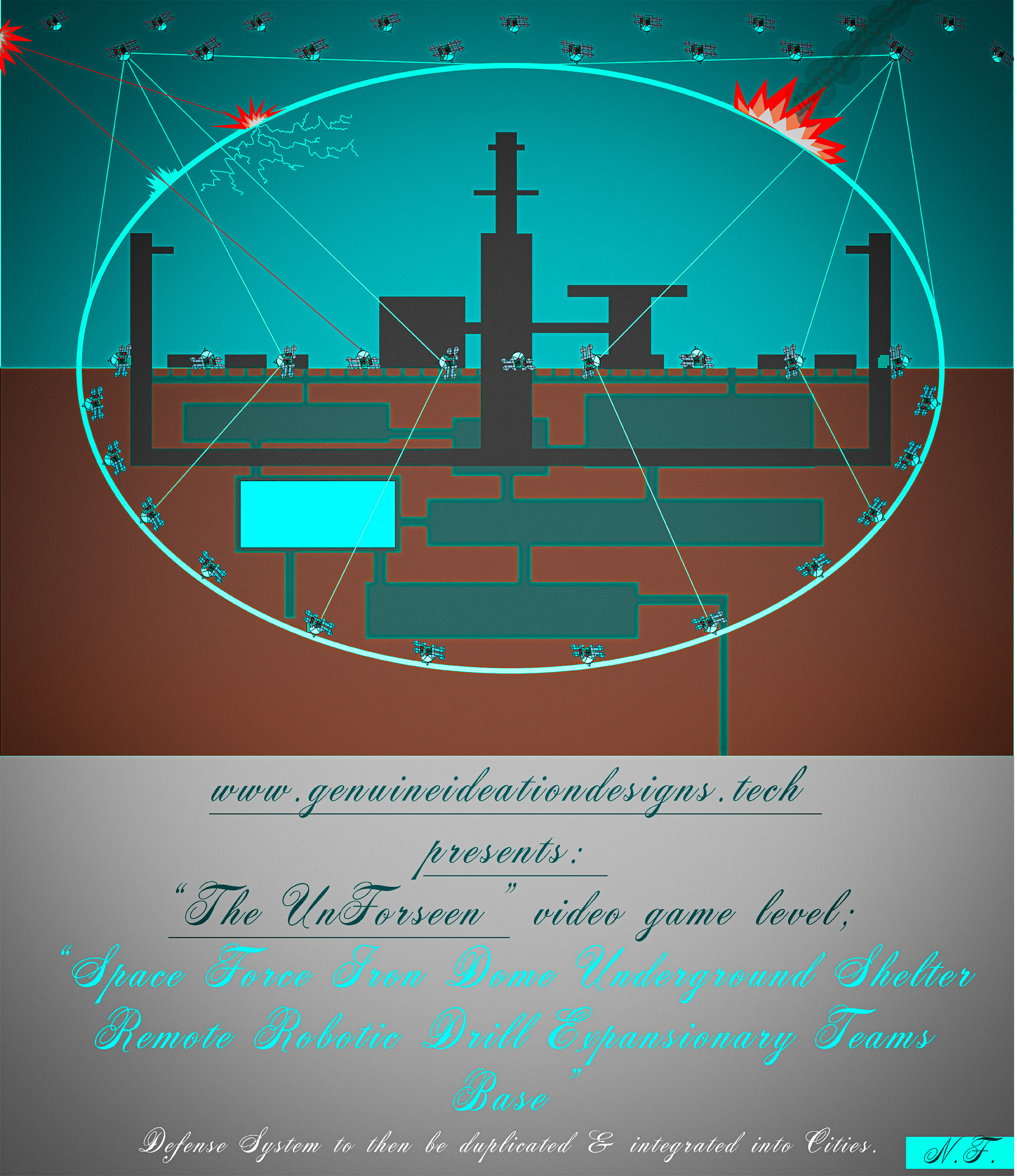
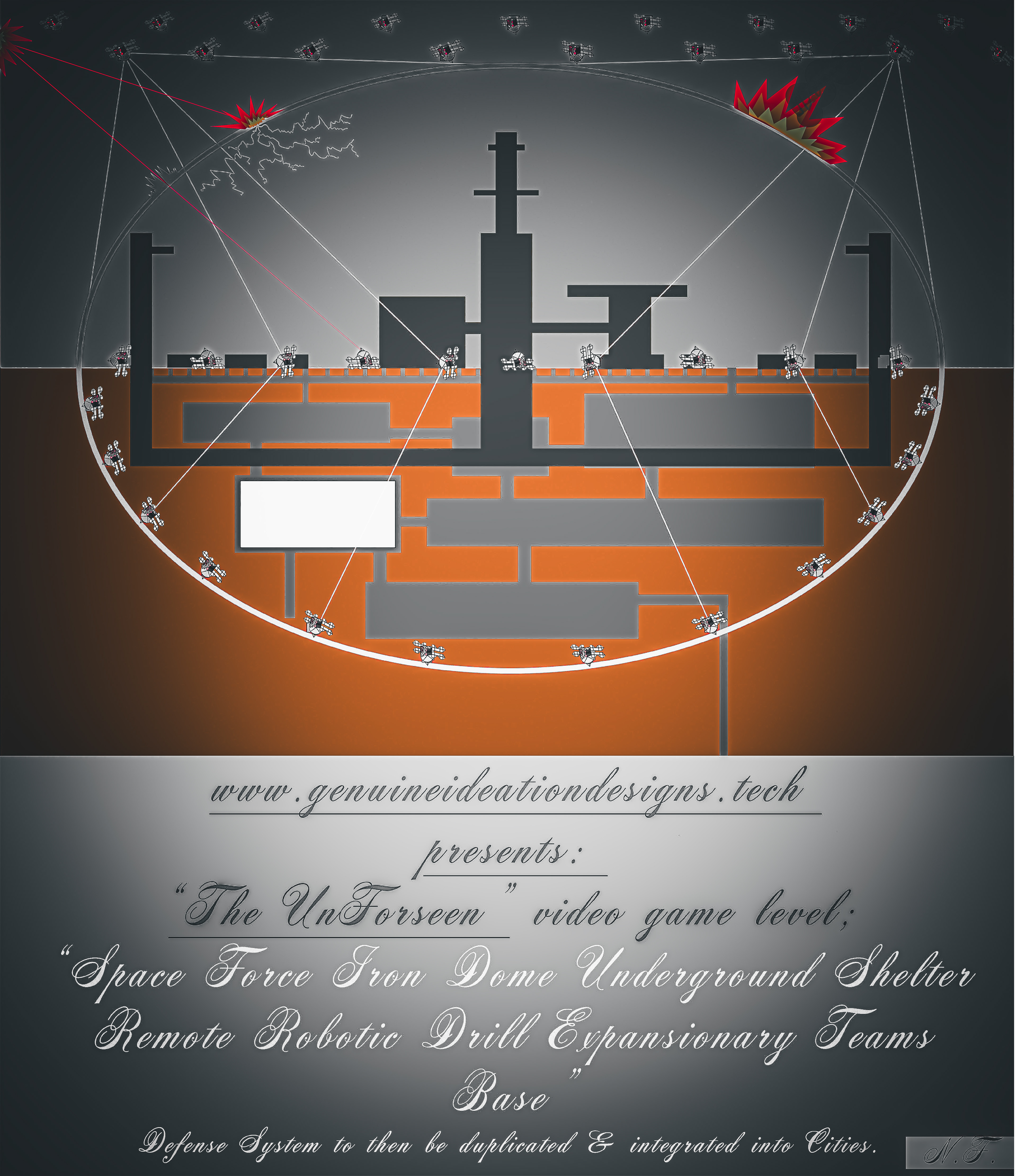
This artwork, attributed to www.genuineideationdesigns.tech, designed by: Nick Folkes, showcases a conceptual design for a video game level titled "The UnForseen." The level is named "Space Force Iron Dome Underground Shelter Remote Robotic Drill Expansionary Teams Base." The design portrays a sophisticated defense system intended for subsequent duplication and integration into urban environments (NF: Once testing & certification in problematic high risk demographics has occured, it will then be copied with room for upgrading and manufactured/ distributed to well liked urban and rural locations.
In those, there are people of high value, they can come from all walks of life, poor or rich, weak or powerful, if you had to choose between all the money or all the weapons and you couldn't have a variation of both, which one would it be?
As long as they are Ladies and Gentleman we won't deactivate the next gen thaad on their precise location & we will embrace them in the community). In it, we will have not for profit military exchange services and same with medical design to testing & certifying into production.
Key elements of the design include:
A central underground shelter with a complex layout, possibly representing various facilities and operational areas.
An encompassing 21st century iron dome, depicted with a luminescent blue outline, suggesting a protective barrier or energy shield.
Numerous robotic units positioned both within and outside the dome, indicating an automated defense or operational network. (NF: wasp loitering swarm drones; (W.L.S.D.'s=(WELL-SAIDS))
The presence of explosive and electrical effects around the dome, possibly representing external threats being neutralized by the defense system.
Strategic placement of beams and pathways, signifying communication and energy transfer between the robotic units and the central base.=underground robotics factory with build, dispense & repair sub sectors, (robotic rapid wasp nest: (R.R.W.N.)) and/or (NF: wasp loitering swarm drones; (W.L.S.D.'s=(WELL-SAIDS))
The entire presentation is meticulously crafted, with a clear emphasis on advanced technology and strategic defense mechanisms, reflecting a futuristic scenario in a gaming environment. The underlying message is to envision a robust defense infrastructure that could be replicated for safeguarding cities (after successful tests in more volatile locations). The signature "N.F." at the bottom right denotes the designer, presumably Nick Folkes.
(2)
The depicted base design, conceptualized for the video game level "The UnForseen" and titled "Space Force Iron Dome Underground Shelter Remote Robotic Drill Expansionary Teams Base," exemplifies a fusion of advanced engineering and cutting-edge scientific principles. The intricate layout and technological elements suggest a highly sophisticated defense and operational hub, with potential real-world applications that could revolutionize urban infrastructure and safety.
Scientific and Engineering Designs:
Iron Dome Technology:
Electromagnetic Shielding: The luminescent blue dome likely represents an advanced electromagnetic shield capable of deflecting and neutralizing external threats, such as projectiles or energy attacks. This technology could be developed using high-intensity plasma fields or superconducting magnets to create a protective barrier.
“FORT. 93Resilience”, + etc.:
By: Nick Folkes
Creating an advanced electromagnetic shield as described involves complex principles from both physics and engineering. Here are some key scientific and mathematical concepts that could be involved:
1. Maxwell's Equations
Maxwell's equations describe the fundamentals of electromagnetism, which would be essential in understanding and designing an electromagnetic shield.
Gauss's Law for Electricity:
∇⋅E=ρϵ0∇⋅E=ϵ0ρ
Gauss's Law for Magnetism:
∇⋅B=0∇⋅B=0
Faraday's Law of Induction:
∇×E=−∂B∂t∇×E=−∂t∂B
Ampère's Law (with Maxwell's addition):
∇×B=μ0J+μ0ϵ0∂E∂t∇×B=μ0J+μ0ϵ0∂t∂E
2. Plasma Physics
High-intensity plasma fields can be used for shielding purposes. Plasma behavior is described by the Magnetohydrodynamic (MHD) equations, which combine Maxwell's equations with the Navier-Stokes equations for fluid dynamics.
Continuity Equation:
∂ρ∂t+∇⋅(ρv)=0∂t∂ρ+∇⋅(ρv)=0
Momentum Equation:
ρ(∂v∂t+v⋅∇v)=−∇p+J×B+∇⋅Tρ(∂t∂v+v⋅∇v)=−∇p+J×B+∇⋅T
Induction Equation:
∂B∂t=∇×(v×B)−∇×(η∇×B)∂t∂B=∇×(v×B)−∇×(η∇×B)
3. Superconducting Magnets
Superconducting materials exhibit zero electrical resistance and expel magnetic fields, which can be used to create strong magnetic fields necessary for an electromagnetic shield.
Critical Field:Hc(T)=Hc0(1−(TTc)2)Hc(T)=Hc0(1−(TcT)2) where Hc0Hc0 is the critical field at absolute zero, and TcTc is the critical temperature.
4. Lorentz Force
The force exerted on a charged particle in an electromagnetic field is given by the Lorentz force equation:
F=q(E+v×B)F=q(E+v×B)
5. Energy Considerations
To maintain the shield, energy input and efficiency are crucial. The energy density uu of an electromagnetic field is given by:
u=12(ϵ0E2+1μ0B2)u=21(ϵ0E2+μ01B2)
Practical Implementation
Plasma Generation: Using devices like tokamaks or stellarators to confine plasma.
Magnetic Confinement: Using superconducting magnets to generate the necessary magnetic fields to contain and shape the plasma.
Control Systems: Feedback mechanisms to dynamically adjust the field parameters in response to external threats.
Example Shield Model
Plasma Shield Equation:
E=−∂A∂t−∇ϕE=−∂t∂A−∇ϕ
where AA is the vector potential and ϕϕ is the scalar potential.
Magnetic Field Generation:
B=∇×AB=∇×A
Shield Intensity Control:
J=σ(E+v×B)J=σ(E+v×B)
where σσ is the conductivity of the plasma.
Combining these principles, an electromagnetic shield can be theoretically designed. The actual construction would require advanced materials, precise control systems, and significant energy resources.
Integrated Defense Systems: The dome incorporates automated defense units positioned at strategic points, suggesting a networked system of sensors and weaponry. These units could employ directed energy weapons (DEWs) such as lasers or railguns for interception and neutralization of incoming threats.
Underground Shelter:
Structural Integrity: The underground base is designed to withstand significant external pressure and impact, possibly through the use of advanced composite materials and geotechnical engineering techniques. This ensures the safety and operational continuity of the base even under extreme conditions.
Resource Management: The layout indicates areas designated for resource storage, energy generation, and living quarters, highlighting a self-sustaining ecosystem. Integration of renewable energy sources, such as geothermal or advanced nuclear reactors, could provide long-term energy solutions.
Remote Robotic Operations:
Automated Drilling and Expansion: The presence of remote robotic drills suggests an autonomous system for expanding and maintaining the base. These robots could be equipped with AI-driven navigation and decision-making capabilities, enabling them to perform complex tasks without human intervention.
Maintenance and Repair: The robots could also serve in repair and maintenance roles, utilizing advanced robotics and nanotechnology to ensure the base remains operational with minimal human oversight.
Future Benefits and Real-World Engineering:
Urban Defense and Safety:
City Protection: Implementing such iron dome systems in urban environments could provide unprecedented protection against aerial threats, including missiles and drones, enhancing the safety of civilian populations.
Disaster Resilience: The underground shelter concept could be adapted for use as disaster relief hubs, providing safe havens during natural disasters or other emergencies.
Sustainable Living and Resource Management:
Self-Sustaining Communities: The design's emphasis on resource management and autonomous operations could pave the way for self-sustaining underground communities, reducing dependency on external supplies and enhancing resilience.
Efficient Resource Utilization: Advanced drilling and resource extraction technologies could enable efficient use of underground resources, supporting sustainable urban expansion without significant environmental impact.
Technological Advancements:
AI and Robotics: The development and deployment of AI-driven robotic systems for maintenance and expansion could lead to breakthroughs in robotics, enhancing automation across various industries.
Advanced Materials and Energy Systems: Research into advanced materials for structural integrity and innovative energy systems could yield new technologies that benefit a wide range of applications, from construction to energy generation.
Engineering into Reality:
To engineer this design into reality, a multidisciplinary approach involving the following steps is required:
Research and Development:
Material Science: Invest in the development of advanced composite materials and superconductors for constructing resilient and efficient structures.
Energy Systems: Develop compact and reliable renewable energy sources, such as small modular reactors or high-efficiency solar panels, to power the base.
Prototype and Testing:
Scale Models: Create scale models and prototypes of the defense systems and robotic units to test their functionality and effectiveness in controlled environments.
Simulation and Modeling: Use advanced computational models to simulate various scenarios, ensuring the design can withstand different types of threats and operational challenges.
Interdisciplinary Collaboration:
Engineering and AI: Foster collaboration between engineers, AI researchers, and robotics experts to integrate advanced automation and (programmed on independent protected networks, that best suits the alliance needs, to protect good humans and to combat evil 'weaponized A.I.' on enemy networks through utilization of 'Friendly A.I.' on 'independant quantum computing networks' seperate from evil networks)
(Where does ai come from? Is it extra terrestrial?)(I firmly believe there is 2 types of ai. The good ai and the evil ai. Some think that’s not possible and that it is all one entity, not separate independent ones) decision-making capabilities into the base's systems.
Urban Planning and Safety: Work with urban planners and safety experts to adapt the design for real-world applications, ensuring it meets the needs of modern cities and their inhabitants.
By leveraging cutting-edge technology and fostering interdisciplinary collaboration, the conceptual design of the "Space Force Iron Dome Underground Shelter Remote Robotic Drill Expansionary Teams Base" could be transformed into a tangible reality, offering enhanced protection, sustainability, and resilience for future urban environments.
(3)
The construction and operation of the "Space Force Iron Dome Underground Shelter Remote Robotic Drill Expansionary Teams Base" would require the implementation of several futuristic engineering techniques. Here are some key techniques and technologies that could be best implemented:
Construction Techniques:
3D Printing and Additive Manufacturing:
Large-Scale 3D Printing: Utilize large-scale 3D printers to construct the underground shelters and structural components. This technology allows for rapid, precise, and cost-effective building, using materials such as concrete, metals, or advanced composites.
Modular Construction: Implement modular construction techniques, where prefabricated sections of the base are manufactured off-site and then assembled on-site. This method reduces construction time and improves quality control.(i.e. Bring in by aircraft to remote isolated mine): could be airlifted to remote isolated locations for the potential of less vulnerability, but is that possible in the 21st century to be safer in remote locations?
Advanced Materials:
Nanomaterials and Smart Materials: Use nanomaterials to create ultra-strong and lightweight structures. Smart materials, which can respond to environmental changes, could be integrated for adaptive structural integrity and self-repairing capabilities.
High-Performance Alloys: Employ high-performance alloys for critical components, ensuring durability and resistance to extreme conditions.
Geotechnical Engineering:
Soil Stabilization: Use advanced soil stabilization techniques to ensure the underground base is structurally sound and resistant to seismic activities. This could include chemical grouting or the use of geosynthetic materials.
Underground Excavation Technologies: Utilize tunnel boring machines (TBMs) and robotic drilling units for precise and efficient excavation. AI-driven TBMs can optimize drilling patterns and minimize environmental impact.
Operational Techniques:
Artificial Intelligence and Machine Learning:
Autonomous Systems: Implement AI for autonomous operation of robotic units, including maintenance, resource extraction, and defense. Machine learning algorithms can optimize performance and adapt to new threats or operational needs.
Predictive Maintenance: Use AI to predict and prevent equipment failures, ensuring continuous operation and reducing downtime. Sensors and IoT devices can monitor the health of various systems in real-time.
Energy Management:
Renewable Energy Integration: Incorporate renewable energy sources, such as advanced solar panels, wind turbines, and geothermal systems, to ensure a sustainable energy supply. Energy storage solutions, like next-generation batteries or supercapacitors, can provide reliable power during peak demands.
Smart Grid Technology: Use smart grid technology to efficiently manage and distribute energy within the base. This system can dynamically allocate resources, reducing waste and optimizing energy usage.
Cybersecurity and Communication:
Quantum Encryption: Implement quantum encryption for secure communication and data protection. This ensures that sensitive information and operational commands are safe from cyber threats.
(Threat example: Cyber Spy Rat Bully Suicide Saboteur:) If they attack good people it is because they hate themselves and need attention. Instead of been a piece of shit to everyone, they should take some good medicine for there ADHD type symptoms, mind there business and develop non-trouble causing hobbies to keep there mind pre-occupied and out of trouble.)
A person who likes themselves does not attack good people, they mind there business even if they are not getting enough attention.)
High-Speed Data Networks: Use high-speed fiber-optic or satellite communication networks to maintain robust and reliable communication channels within the base and with external entities.(Imperative for re-enforcements, rescue operations.)
Advanced Robotics:
Swarm Robotics: Deploy swarm robotics for coordinated tasks, such as defense, maintenance, and exploration. These robots can work together autonomously, adapting to new challenges and optimizing task execution. (If enemy networks weaponized ai is created, there must be a kill switch (Theoretically) that we can activate or a methodology of some other kind to do so.)
Humanoid and Specialized Robots: Use humanoid robots for tasks requiring human-like dexterity and specialized robots for specific functions, such as heavy lifting, precision drilling, or hazardous material handling.
Implementation Strategies:
Interdisciplinary Collaboration:
Collaborate with Experts: Work with experts in AI, robotics, materials science, and geotechnical engineering to integrate cutting-edge technologies effectively.
Cross-Disciplinary Research: Foster cross-disciplinary research to innovate and develop new techniques tailored to the base's unique requirements.
Prototyping and Simulation:
Digital Twins: Create digital twins of the base to simulate and optimize construction and operational processes.(100-1000 developer teams creating different style video games of original blueprint design to broaden perspective, then choose best ones, i.e. top 5) This allows for testing and refining systems in a autocad scaling type virtual environment before implementation into a physical one in a volatile zone to be then integrated into Urban & Rural settings.
Prototyping: Develop prototypes of critical systems and components to validate performance and identify potential improvements.
Regulatory and Safety Compliance:
Adhere to Regulations: Ensure all construction and operational activities comply with relevant safety and regulatory standards.
Safety Protocols: Develop comprehensive safety protocols and emergency response plans to protect personnel and infrastructure.
By integrating these futuristic engineering techniques, the construction and operation of the "Space Force Iron Dome Underground Shelter Remote Robotic Drill Expansionary Teams Base" can be realized, offering enhanced protection, sustainability, and resilience in both virtual and real-world applications.
=======================================
#(6):
"Base 21st Alliance":
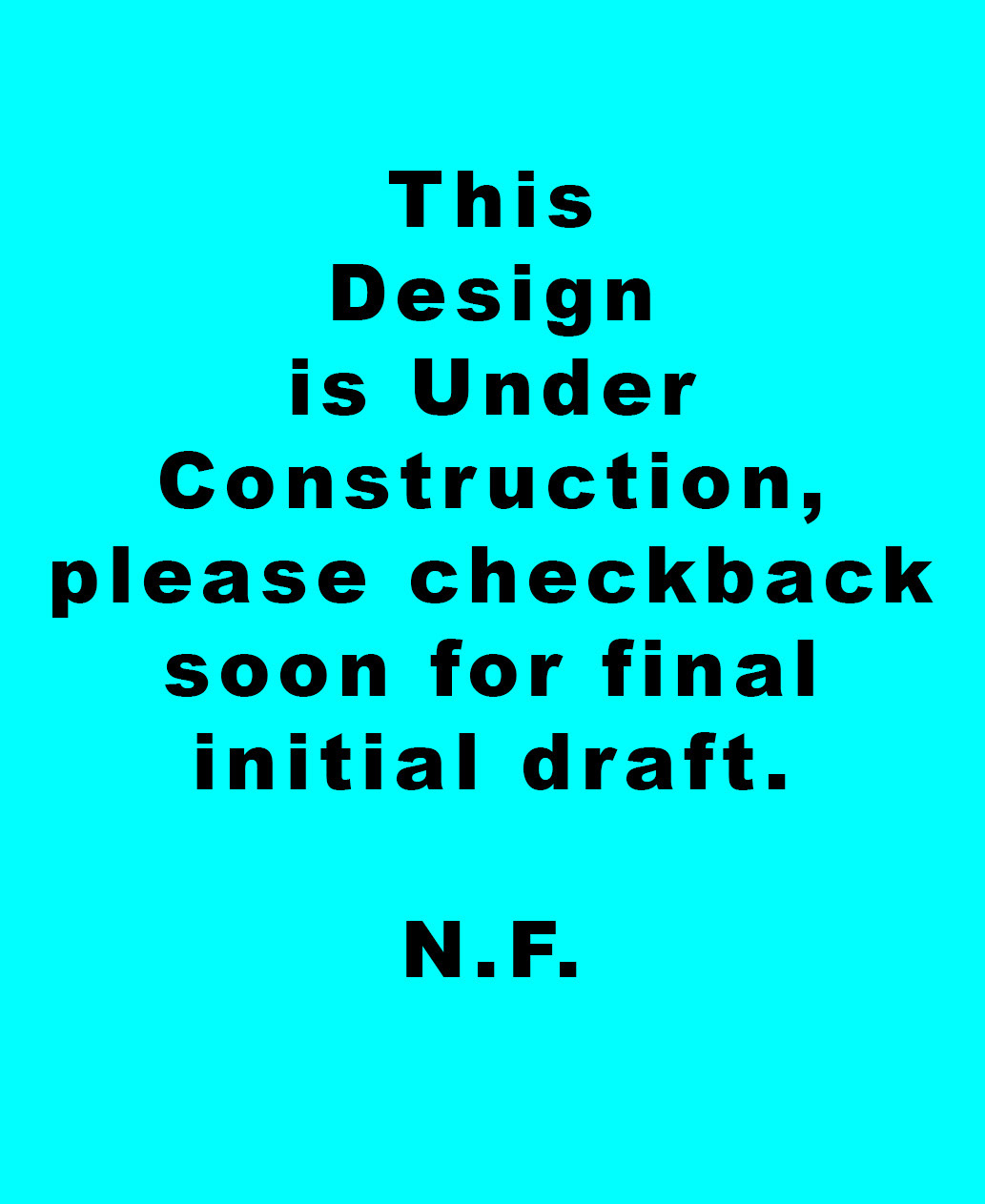
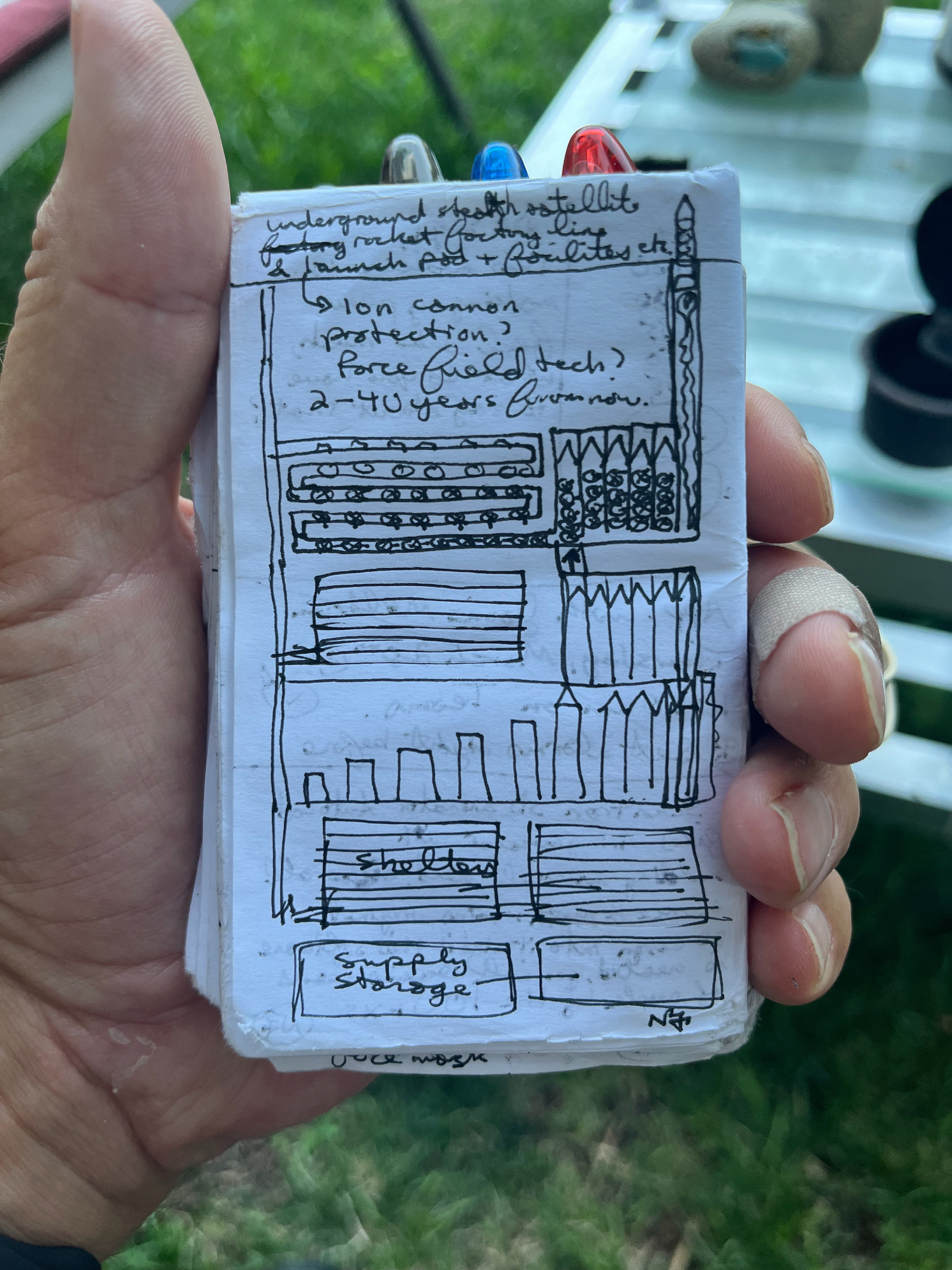
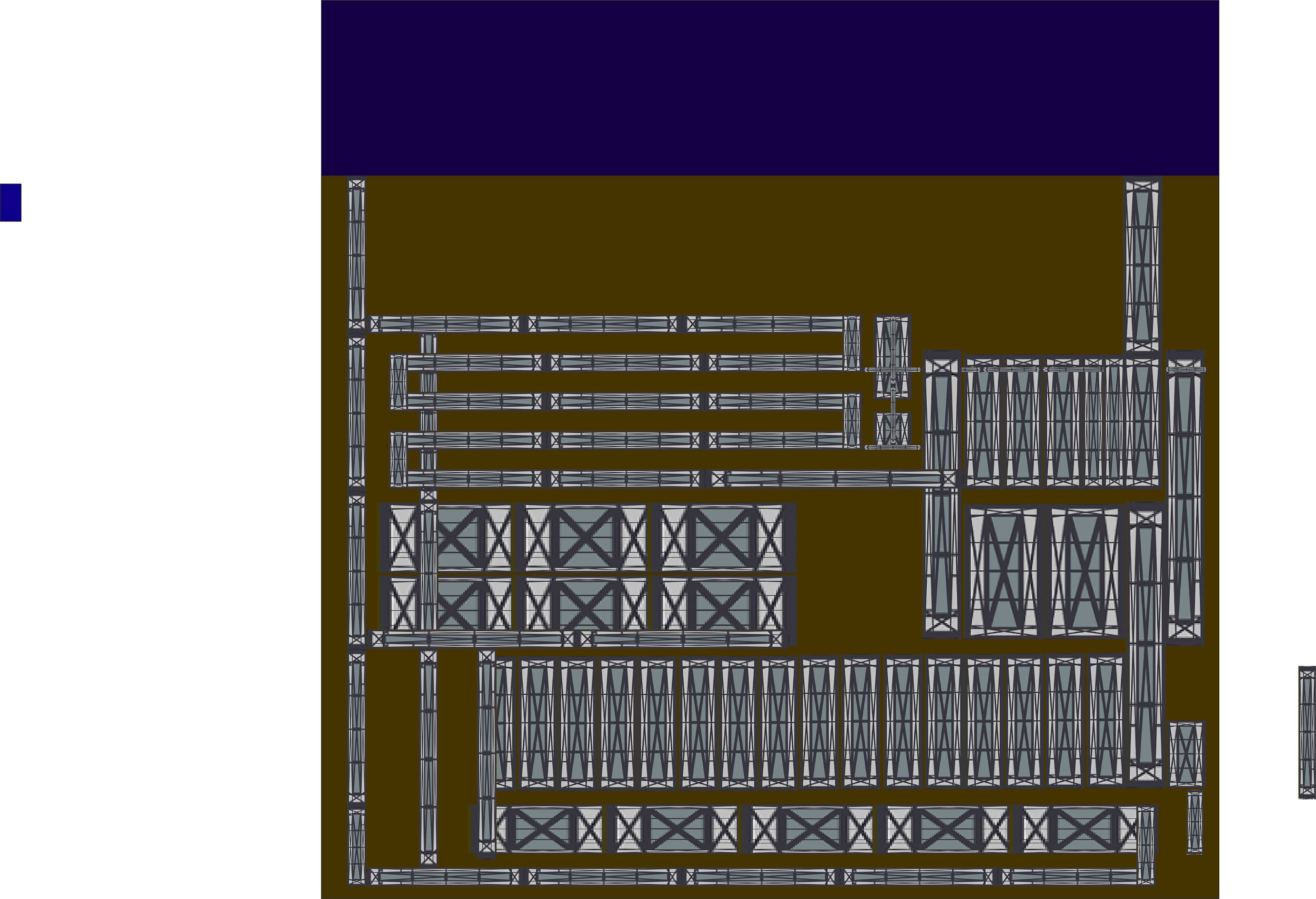
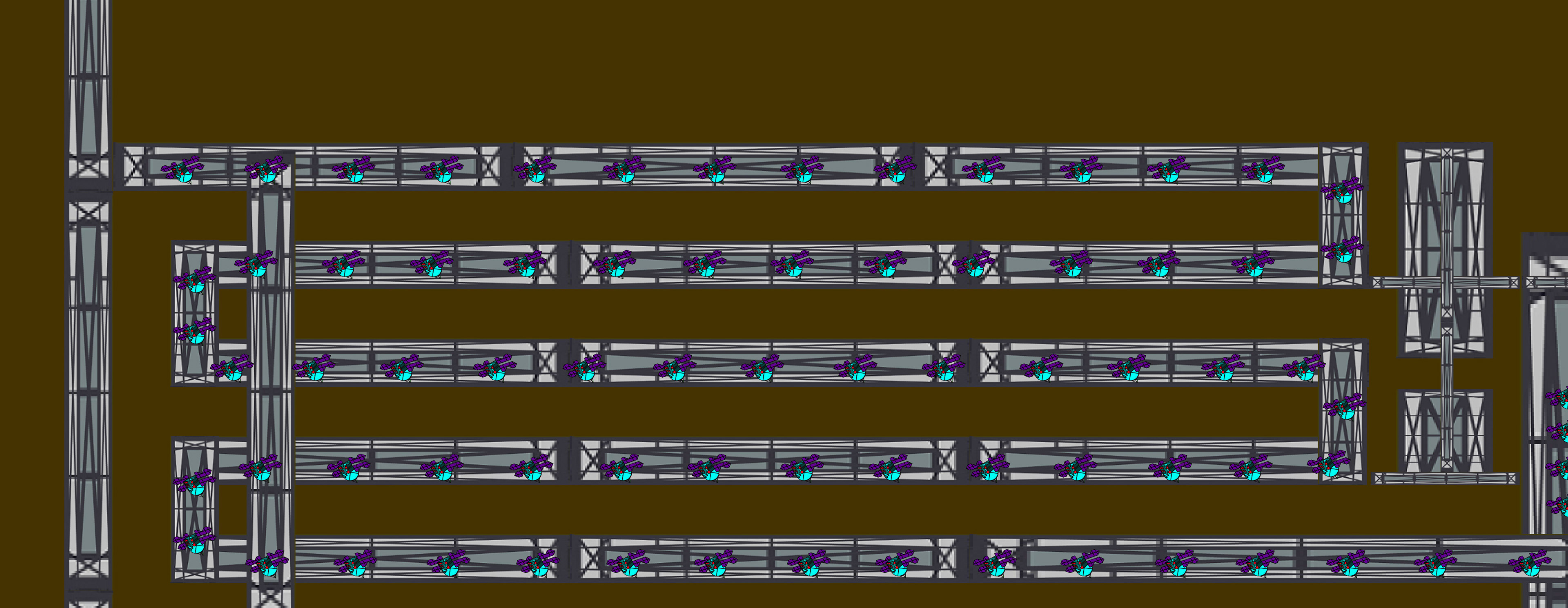
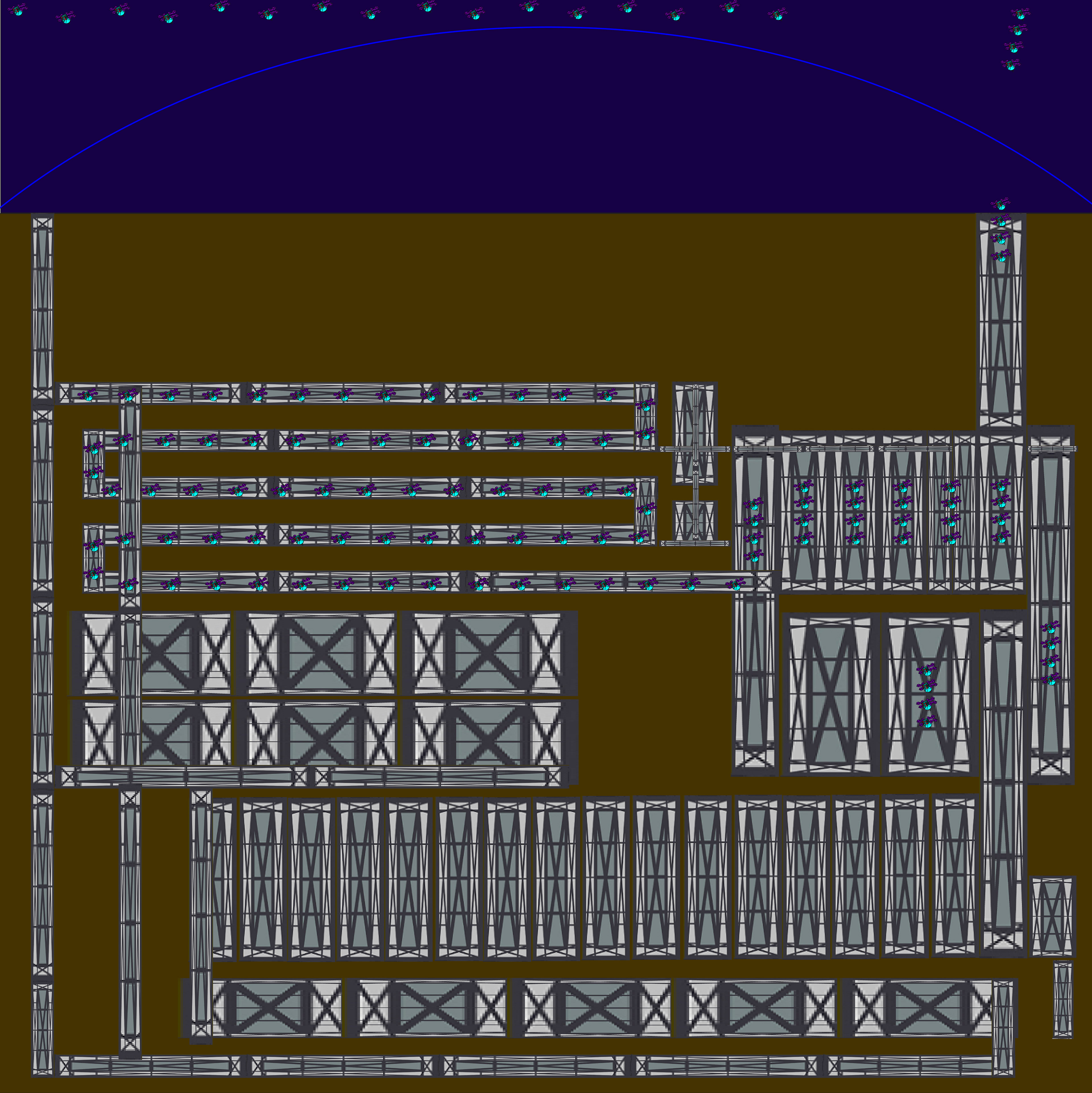
"Base 21st Alliance"
enhanced a.i. description Coming soon!!! stay tuned.
=============================================================================================
#(3):
One of the last Scouts:
((Volunteer Fire Fighting Design)
(F.C.C.) Fire Crisis Combat);
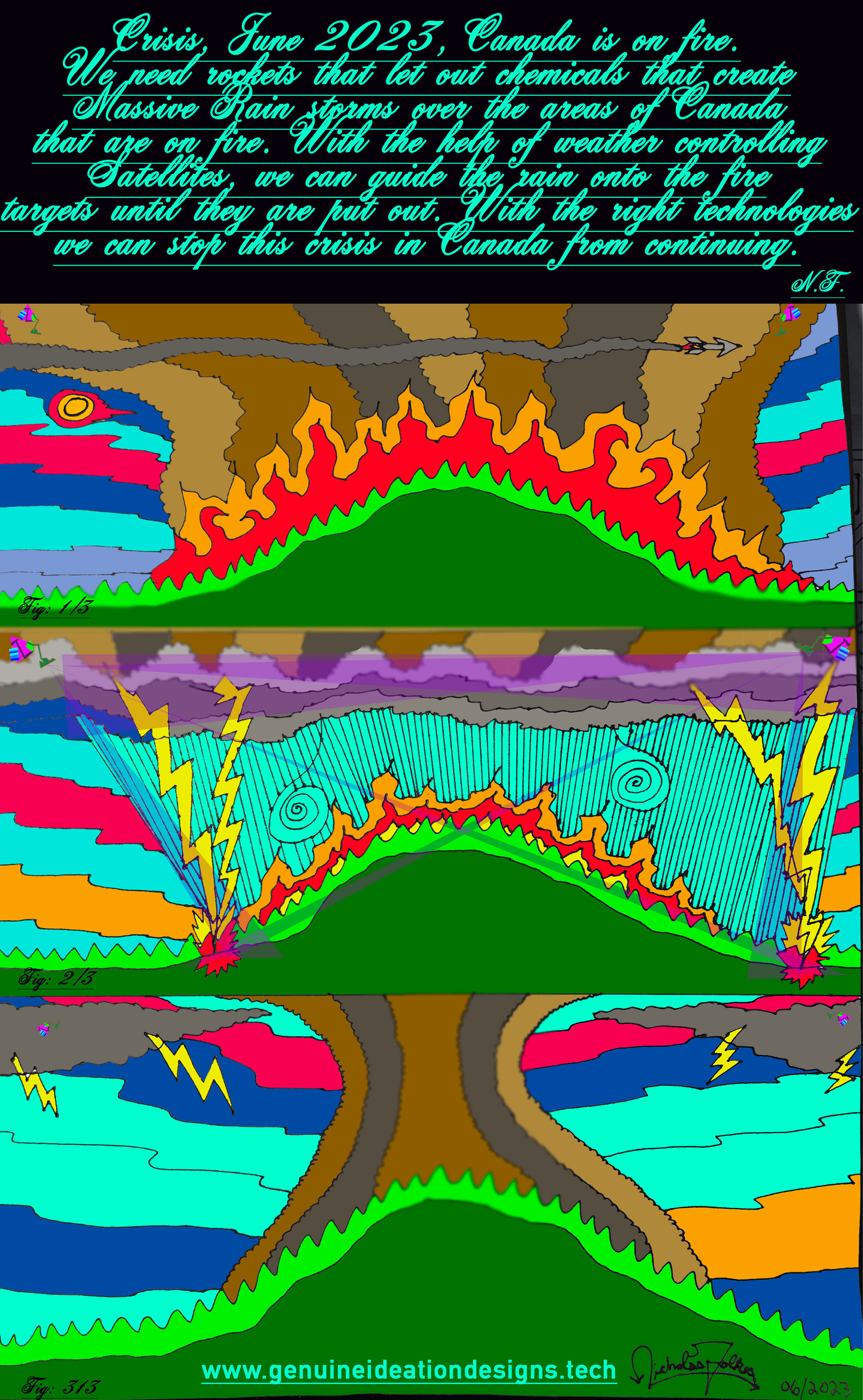
To describe the technologies and engineering required to make the concept in the artwork by Nick Folkes a reality, we need to focus on several key areas:
1. Satellite-Based Weather Control
Technologies:
Advanced Satellites: Equip satellites with sensors to monitor weather patterns, detect fires, and control precipitation.
AI and Machine Learning: Use AI algorithms to predict fire spread and optimal locations for inducing rainfall.
Communication Systems: Develop robust communication systems to relay real-time data and commands between ground stations and satellites.
Engineering:
Satellite Design: Design satellites capable of withstanding the harsh conditions of space while carrying sophisticated sensors and communication equipment.=Advancing Force Field tech.
Orbital Mechanics: Plan satellite orbits to maximize coverage and efficiency in monitoring and responding to fires.
2. Chemical Rain Induction Rockets
Technologies:
Chemical Compounds: Research and develop safe, effective chemical compounds that can induce rainfall when released into the atmosphere.
Rocket Propulsion: Develop rockets capable of carrying these compounds to the required altitudes and releasing them precisely.
Weather Simulation Models: Use advanced weather simulation models to predict the effects of these chemicals and optimize their use.
Engineering:
Rocket Design: Design rockets with the capability to accurately deliver chemical payloads to specific altitudes and locations.
Payload Delivery Systems: Engineer systems to release chemical compounds in a controlled and effective manner.
3. Fire Detection and Monitoring
Technologies:
Remote Sensing: Use infrared and thermal imaging sensors on satellites and drones to detect fires early and monitor their spread.
Geospatial Analysis: Employ GIS (Geographic Information Systems) to analyze and visualize fire data.
IoT Sensors: Deploy ground-based IoT sensors to provide real-time data on temperature, humidity, and fire presence.
Engineering:
Sensor Integration: Integrate various sensors into a cohesive system for comprehensive fire monitoring.
Data Processing: Develop systems for processing and analyzing the vast amounts of data generated by these sensors in real time.
4. Coordination and Response
Technologies:
Command and Control Centers: Establish centralized command centers to coordinate the deployment of rain inducing rockets and monitor satellite data.
Autonomous Drones: Use drones for close-range monitoring and to assist in firefighting efforts.
Cloud Computing: Leverage cloud computing for data storage, processing, and access by multiple stakeholders.
Engineering:
Network Infrastructure: Build resilient and high-speed network infrastructure to support real-time data transfer and communication.
Control Algorithms: Develop algorithms for automated decision-making and coordination of response efforts.
5. Environmental and Safety Considerations
Technologies:
Eco-friendly Chemicals: Ensure the chemicals used for rain induction are environmentally safe and do not harm the ecosystem.
Safety Protocols: Develop protocols to ensure the safety of populations and ecosystems when deploying these technologies.
Engineering:
Risk Assessment: Conduct thorough risk assessments to understand and mitigate potential adverse effects.
Regulatory Compliance: Ensure all technologies and methods comply with national and international regulations.
Implementation Plan
Research and Development: Invest in R&D to advance satellite, rocket, and chemical technologies.
Pilot Projects: Conduct pilot projects in controlled environments to test and refine the technologies.
Infrastructure Development: Build the necessary infrastructure for satellite launches, rocket deployments, and data processing.
Training and Education: Train personnel in the use of these advanced technologies and establish educational programs for continuous learning.
Public-Private Partnerships: Foster collaborations between governments, private companies, and research institutions to share knowledge, resources, and funding.
By integrating these advanced technologies and engineering solutions, we can turn the concept in Nick Folkes' artwork into a feasible reality, helping to mitigate the impact of wildfires and protect ecosystems.
2)
what are the most advanced compounds are necessary to make the best type of satellite controlling chemical rain induction rockets to stop forest fires?
To create the most advanced and effective chemical rain induction rockets for satellite-controlled deployment to stop forest fires, the compounds used must have specific properties that enable them to induce precipitation reliably and safely. Here are some of the most advanced compounds that could be used:
1. Silver Iodide (AgI)
Properties: Silver iodide has been used for cloud seeding for decades. It serves as an effective nucleation agent that promotes the formation of ice crystals in supercooled clouds, leading to precipitation.
Advantages: Proven effectiveness, relatively low cost, and well-understood mechanisms.
Challenges: Environmental concerns about the potential toxicity of silver accumulation.
2. Potassium Iodide (KI)
Properties: Potassium iodide is another commonly used cloud-seeding agent. It acts similarly to silver iodide but is considered less toxic.
Advantages: Effective in nucleating cloud droplets, less environmental impact compared to silver iodide.
Challenges: Requires precise conditions for optimal effectiveness.
3. Calcium Chloride (CaCl2)
Properties: Calcium chloride is a hygroscopic compound that can absorb moisture from the air and induce precipitation.
Advantages: Readily available, relatively safe, and can be effective in various weather conditions.
Challenges: May require large quantities to be effective, potential for soil and water contamination if not managed properly.
4. Sodium Chloride (NaCl)
Properties: Common table salt can be used to enhance precipitation by increasing the size and weight of cloud droplets.
Advantages: Abundant and inexpensive, effective in a variety of cloud types.
Challenges: Potential for environmental impact due to salt buildup.
5. Liquid Propane
Properties: When released into supercooled clouds, liquid propane can cause rapid freezing, leading to the formation of ice crystals and subsequent precipitation.
Advantages: Highly effective in specific weather conditions, can produce immediate results.
Challenges: Handling and storage require careful management due to its gaseous state at ambient temperatures.
6. Urea
Properties: Urea can act as a cloud condensation nuclei (CCN) enhancer, promoting the growth of water droplets in clouds.
Advantages: Biodegradable and environmentally friendly.
Challenges: Effectiveness can vary based on cloud composition and ambient conditions.
Advanced Compounds and Research
7. Nano-Structured Ice Nucleating Agents
Properties: These are engineered particles designed at the nanoscale to optimize ice nucleation efficiency.
Advantages: Can be tailored to specific weather conditions and cloud types, potentially higher effectiveness than traditional agents.
Challenges: High cost of production, need for extensive testing to ensure environmental safety.
8. Graphene Oxide
Properties: Recent research suggests that graphene oxide can enhance cloud seeding by acting as effective CCN.
Advantages: High surface area and hydrophilicity, potential for highly efficient nucleation.
Challenges: Environmental and health impact of widespread graphene use is still under investigation.
Implementation Strategy
Research and Testing: Conduct extensive laboratory and field tests to determine the effectiveness and safety of each compound under various atmospheric conditions.
Environmental Impact Assessment: Evaluate the long-term environmental impact of the compounds to ensure they do not harm ecosystems or human health.
Optimized Delivery Systems: Develop advanced delivery mechanisms for rockets to release these compounds at precise altitudes and locations.
Regulatory Approval: Work with environmental and regulatory agencies to obtain approval for the use of these compounds in cloud seeding.
Continuous Monitoring: Implement monitoring systems to track the environmental impact and effectiveness of cloud seeding efforts.
By leveraging these advanced designs by Nick Folkes for compounds and integrating them with cutting-edge satellite and rocket technologies, it is possible to develop a highly effective system for inducing rain and combating forest fires.
=============================================================================================
#(7):
presidential/ V.I.P.: Protective oxygen & air conditioned mobile stage case;
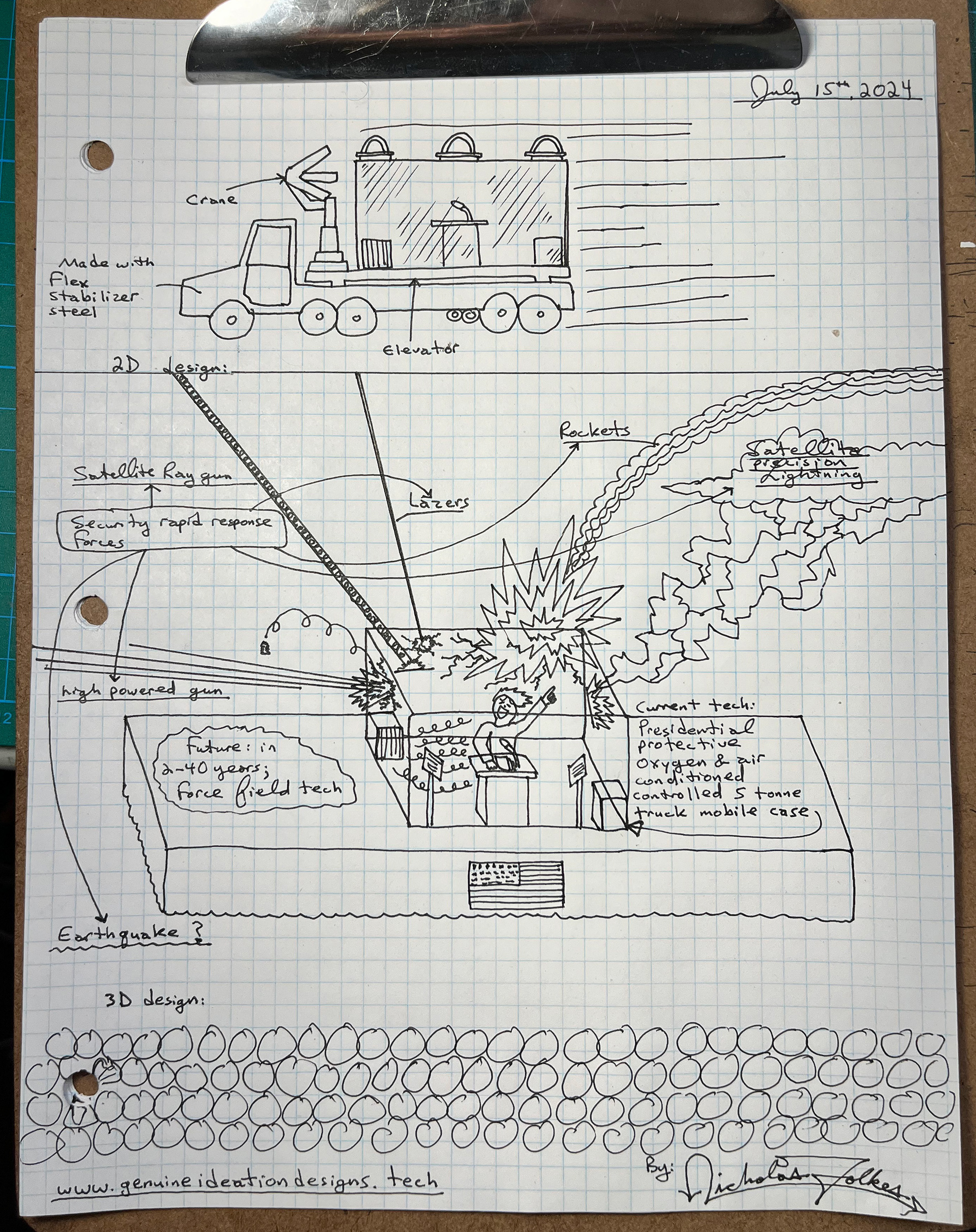
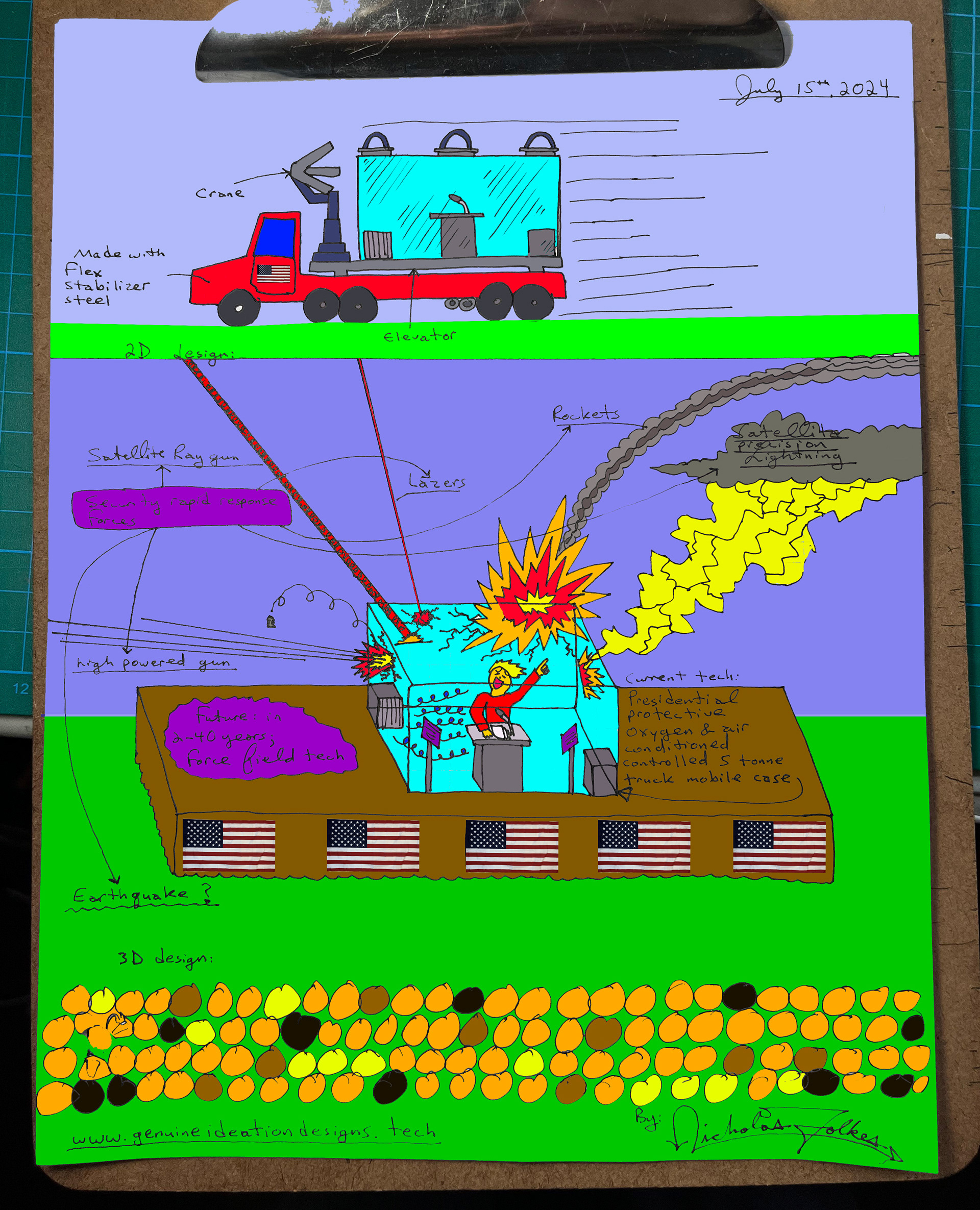
i)
To create a comprehensive analysis and development plan for the protective case for presidential candidates as illustrated in Nick Folkes' drawing, we need to break down the components and address each aspect scientifically and mathematically. Here’s a detailed approach:
1. Structure and Materials
Flex Stabilizer Steel:
Material Strength: Calculate the tensile strength, yield strength, and impact resistance of Flex Stabilizer Steel. Use the formula:σ=FAσ=AF where σσ is the stress, FF is the force, and AA is the area.
Stress Analysis: Conduct finite element analysis (FEA) to simulate stress distribution and deformation under various load conditions (impacts, explosions, etc.).
Crane and Elevator System:
Load Calculations: Determine the load-bearing capacity using:W=F⋅dW=F⋅d where WW is the work done, FF is the force, and dd is the distance moved by the elevator.
Motor Requirements: Calculate the power requirements for the crane and elevator motors using:P=WtP=tW where PP is power, WW is work, and tt is time.
2. Defense Mechanisms
Rockets and Lasers:
Projectile Motion: Use kinematic equations to determine the trajectory of rockets:x(t)=v0xt+12axt2x(t)=v0xt+21axt2y(t)=v0yt+12ayt2y(t)=v0yt+21ayt2 where x(t)x(t) and y(t)y(t) are the horizontal and vertical positions, v0xv0x and v0yv0y are initial velocities, and axax and ayay are accelerations.
Laser Efficiency: Calculate energy efficiency and heat dissipation using:η=PoutPinη=PinPout where ηη is efficiency, PoutPout is output power, and PinPin is input power.
High-Powered Gun:
Ballistics: Calculate the muzzle velocity and impact force using:F=m⋅aF=m⋅a where FF is force, mm is mass, and aa is acceleration.
3. Environmental Protection
Presidential Protective Case:
Air Filtration and Conditioning: Calculate the required air exchange rate and filter efficiency to ensure a safe environment:Q=V⋅ACH60Q=V⋅60ACH where QQ is the flow rate, VV is the volume of the case, and ACHACH is air changes per hour.
Soundproofing: Determine the material’s sound transmission class (STC) and its effectiveness in reducing noise.
4. Additional Elements
Future Technology Integration:
Force Field Tech: Investigate the feasibility of developing a force field using electromagnetic fields or plasma.
Satellite Raygun: Examine the potential for satellite-based defense systems using directed energy weapons.
Security Rapid Response Forces:
Deployment Strategy: Design a rapid deployment system using drones and automated response units.
Communication Systems: Develop secure, encrypted communication channels for real-time coordination.
5. Mathematical and Scientific Justifications
Stress and Impact Resistance:
Finite Element Analysis (FEA): Conduct simulations to predict the performance of materials under stress.
Impact Testing: Perform empirical tests to validate the simulation results.
Energy Calculations for Defense Systems:
Energy Density: Calculate the energy density required for lasers and rockets.
Thermal Management: Design cooling systems to manage heat generated by defense mechanisms.
6. Advanced Enhanced Literacy Descriptions
Technical Documentation:
Create detailed blueprints with annotations explaining each component's function.
Develop a user manual for operating the protective case, including emergency procedures and maintenance guidelines.
7. Investor Proposal
Executive Summary:
Highlight the innovative features and potential market for the protective case.
Provide a cost-benefit analysis, emphasizing the safety and security benefits for high-profile individuals.
Technical Appendices:
Include detailed engineering drawings, material specifications, and scientific calculations.
Present case studies and simulations demonstrating the effectiveness of the design.
This comprehensive approach ensures the protective case is both scientifically robust and practical, ready for manufacturing and deployment.
ii)
Detailed Calculations and Specifications for the Protective Case
1. Structure and Materials
Material Selection:
Flex Stabilizer Steel:Yield Strength Calculation:σy=FyAσy=AFy where FyFy is the yield force and AA is the cross-sectional area.
Typical yield strength for high-strength steel is about 250-500 MPa (megapascals).
Bulletproof Glass:Thickness Determination:t=v2⋅ρ2⋅σt=2⋅σv2⋅ρ where tt is the thickness, vv is the bullet velocity, ρρ is the density of the glass, and σσ is the tensile strength.
Standard bulletproof glass has a density of about 2.5 g/cm³ and can stop bullets from handguns with a velocity up to 1500 m/s.
Composite Materials:
Layered Composites: Combining materials like Kevlar, aramid fibers, and ceramic plates to enhance protection.
Energy Absorption:E=∫0dF(x) dxE=∫0dF(x)dx where EE is the energy absorbed, F(x)F(x) is the force as a function of distance xx, and dd is the penetration depth.
Construction Methods:
Welding and Riveting: Use high-strength welding techniques and rivets for joining steel plates.
Lamination: Laminate bulletproof glass layers with polycarbonate to increase impact resistance.
Composite Layering: Apply layered composites in critical areas to absorb and dissipate energy from impacts.
2. Defense Mechanisms
Rocket and Laser Defense Systems:
Rocket Launchers:Propellant Force Calculation:F=m⋅ΔvΔtF=Δtm⋅Δv where mm is the mass of the rocket, ΔvΔv is the change in velocity, and ΔtΔt is the time interval.
For a 10 kg rocket achieving a velocity of 300 m/s in 0.5 seconds:
F=10⋅3000.5=6000 NF=0.510⋅300=6000N
Laser Systems:Energy Requirements:E=P⋅tE=P⋅t where EE is the energy, PP is the power output, and tt is the time.
For a 10 kW laser operating for 5 seconds:
E=10,000⋅5=50,000 JE=10,000⋅5=50,000J
High-Powered Gun:
Muzzle Velocity Calculation:v=2⋅Emv=m2⋅E where vv is the muzzle velocity, EE is the kinetic energy, and mm is the mass.
For a bullet with mass 0.02 kg and kinetic energy 2000 J:
v=2⋅20000.02=447.21 m/sv=0.022⋅2000=447.21m/s
3. Environmental Protection
Air Filtration and Conditioning:
Air Exchange Rate:
Q=V⋅ACH60Q=V⋅60ACH
where QQ is the flow rate (m³/h), VV is the volume (m³), and ACHACH is air changes per hour.
For a volume of 30 m³ and 10 air changes per hour:
Q=30⋅1060=5 m³/hQ=30⋅6010=5m³/h
Filter Efficiency:
Use HEPA filters with efficiency up to 99.97% for particles 0.3 microns or larger.
η=Cin−CoutCin×100η=CinCin−Cout×100
where ηη is the efficiency, CinCin and CoutCout are the concentrations of contaminants inside and outside the filter.
Soundproofing:
Sound Transmission Class (STC):
Calculate STC based on the material's ability to attenuate sound:
STC=10log10(PincidentPtransmitted)STC=10log10(PtransmittedPincident) where PincidentPincident is the incident sound power, and PtransmittedPtransmitted is the transmitted sound power.
4. Advanced Enhanced Literacy Descriptions
Technical Documentation:
Blueprints:
Detailed technical drawings with dimensions, materials, and assembly instructions.
User Manual:Comprehensive guide including:
Setup and Operation: Step-by-step instructions for setting up and operating the protective case.
Safety Procedures: Emergency protocols and safety measures.
Maintenance Schedule: Regular maintenance tasks to ensure optimal performance.
Additional Elements to Enhance Design
Active Defense Systems:
Drones: Deploy drones equipped with surveillance and defensive capabilities.
Automated Turrets: Integrate automated turrets with facial recognition and target tracking.
Communication Systems:
Encrypted Channels: Use advanced encryption standards (AES-256) for secure communication.
Redundant Systems: Implement redundant communication systems to ensure uninterrupted connectivity.
Mobility Enhancements:
Hydraulic Suspension: Improve mobility over rough terrain with advanced suspension systems.
Self-Driving Capabilities: Integrate autonomous driving technology for rapid relocation.
Investor Proposal
Executive Summary:
Highlight the innovative aspects, market need, and potential applications.
*****Emphasize safety, security, and technological advancements.*****
Cost-Benefit Analysis:
Initial Investment: Estimate the cost of development and manufacturing.
*****Long-Term Benefits: Project potential savings from prevented threats and enhanced security.*****
Technical Appendices:
Include detailed engineering drawings, material specifications, and empirical test results.
Provide case studies and simulations demonstrating the effectiveness of the protective case.
This detailed approach ensures a scientifically and technically sound design for the protective case, addressing all critical aspects to protect high-profile individuals effectively.
==============================================
==============================================
#(5):
"Base 93-Resilience":
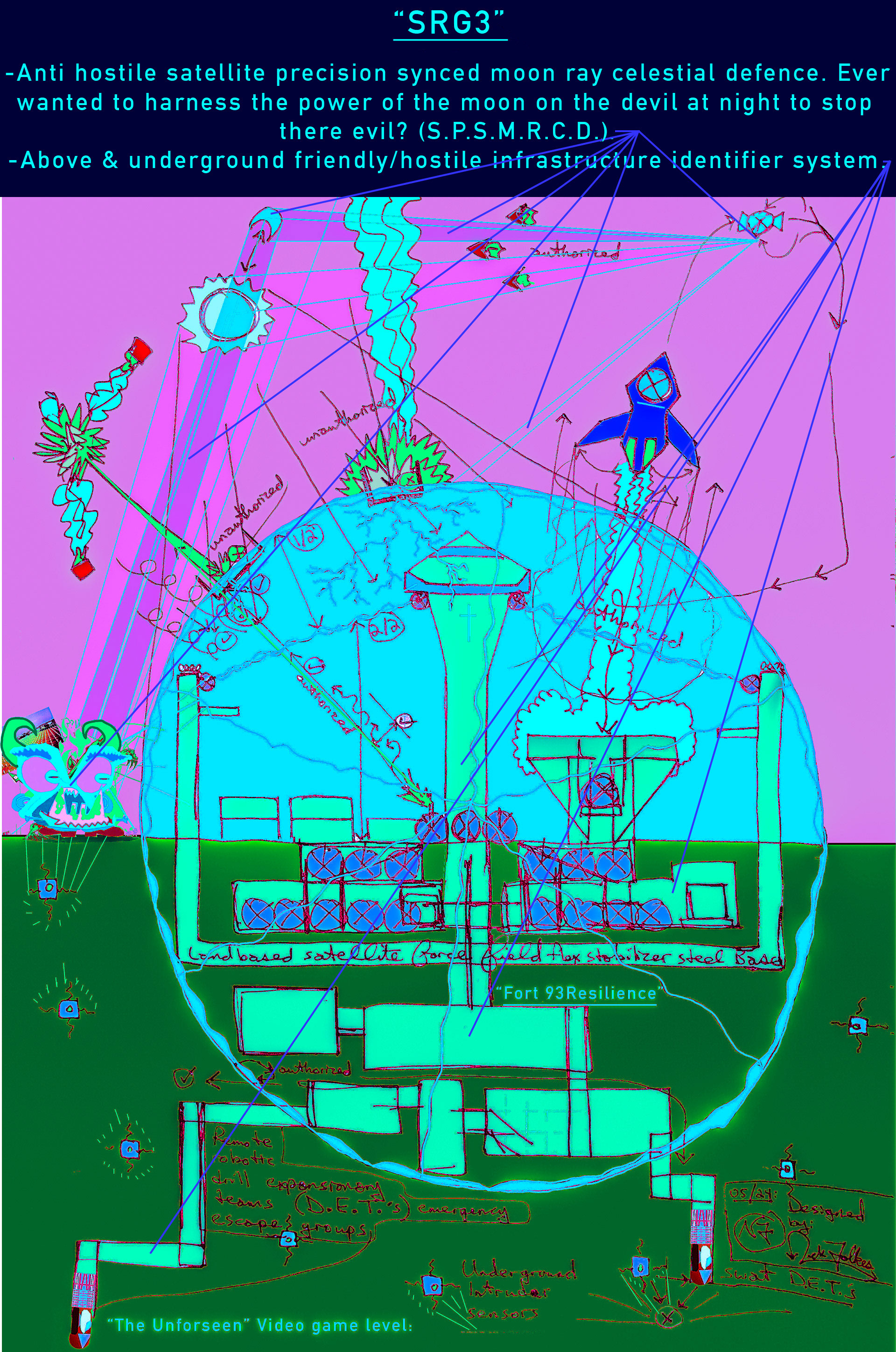
‘The SRG3 Moon Ray” + advanced upgrades
Part. i/ii Batch
To conceptualize and engineer the "SRG3" design by Nick Folkes, a multidisciplinary approach incorporating advanced engineering, security systems, scientific principles, and mathematical equations is necessary. Below is a high-tech, advanced description of the requirements:
Engineering Requirements:
Satellite Technology:
Precision Guidance Systems: Development of algorithms for precision targeting and synchronization with lunar cycles.
Solar Power Harvesting: Utilization of solar panels on satellites to harness energy for moon ray deflection.
Orbital Mechanics: Calculations to ensure optimal satellite positioning for continuous lunar exposure.
Defense Infrastructure:
Fortifications: Design of underground bunkers with reinforced steel and advanced materials to withstand attacks.
Ray Deflection Mechanisms: Engineering of ground-based and satellite-based systems for directing lunar rays.
Autonomous Drones: Deployment of aerial and underground drones for surveillance and defense, equipped with AI for decision-making.
Construction Materials:
Advanced Alloys: Use of lightweight, high-strength alloys for structural integrity.
Smart Materials: Integration of materials with self-healing and adaptive properties for enhanced durability.
Security Systems:
Intrusion Detection:
Sensors: Deployment of high-sensitivity sensors to detect unauthorized entry both above and below ground.
Machine Learning Algorithms: Implementation of AI to analyze sensor data and predict potential threats.
Encryption Protocols:
Quantum Encryption: Use of quantum cryptography to secure communications between satellites and ground stations.
Blockchain Technology: Implementation for secure, tamper-proof logging of all security events.
Countermeasures:
EMP Shields: Installation of electromagnetic pulse shields to protect electronics from hostile attacks.
Laser Defense Systems: Development of ground-based laser systems for neutralizing incoming threats.
Scientific Principles:
Lunar Ray Dynamics:
Reflective Properties: Study of lunar surface reflectivity and its interaction with various wavelengths.
Ray Concentration Techniques: Research on focusing and amplifying lunar rays using satellite mirrors.
Energy Conversion:
Photonics: Exploration of photonic crystals and other materials to enhance energy conversion efficiency.
Thermodynamics: Application of thermodynamic principles to manage heat dissipation in satellite systems.
Astrophysics:
Gravitational Effects: Calculation of gravitational influences on satellite orbits and ray trajectories.
Lunar Phases: Synchronization of defense mechanisms with lunar phases for maximum effectiveness.
Mathematical Equations and Literacy:
Orbital Mechanics:
Kepler's Laws: T2∝R3T2∝R3 to determine satellite orbits.
Newton's Law of Gravitation: F=Gm1m2r2F=Gr2m1m2 for gravitational force calculations.
Energy Calculations:
Photon Energy: E=hνE=hν, where hh is Planck's constant and νν is frequency.
Thermal Dynamics: Q=mcΔTQ=mcΔT for heat transfer in materials.
Signal Processing:
Fourier Transforms: For analyzing and filtering signal data from sensors.
Cryptographic Algorithms: RSA, AES for secure data transmission.
Literary Descriptions:
Technical Manuals: Comprehensive documentation of system designs, including schematics and operational guidelines.
Scientific Papers: Publications detailing the research and development of new materials, energy systems, and security protocols.
Training Guides: Instructional materials for personnel operating and maintaining the defense systems.
Conclusion:
The realization of Nick Folkes' "SRG3" design requires an interdisciplinary approach, integrating cutting-edge engineering, robust security measures, advanced scientific research, and complex mathematical modeling. The collaboration of experts in aerospace, materials science, computer science, and physics is essential to develop and deploy this sophisticated defense system.
----------------------------------------------------------
Part. ii/ii Batch
Advanced spectors:
To develop technology capable of seeing through any ground to any depth and identify friendly or hostile actors, a combination of advanced engineering, security protocols, scientific principles, mathematical equations, and technological innovations is required. Below is a detailed description of these components:
Engineering Requirements:
Ground Penetrating Radar (GPR):
High-Frequency Transmitters and Receivers: Development of high-power, high-frequency radar systems capable of penetrating deep underground layers.
Antenna Arrays: Design of advanced antenna arrays for improved signal resolution and depth penetration.
Signal Processing Units: Creation of fast and efficient signal processing units to interpret radar data in real-time.
Seismic Imaging:
Seismic Sensors: Deployment of a network of sensitive seismic sensors to detect and interpret vibrations caused by movement underground.
Data Integration Systems: Systems for integrating seismic data with GPR data to enhance accuracy.
Magnetotellurics:
Magnetic Field Sensors: Use of sensors to measure natural variations in the Earth's magnetic field caused by subsurface structures.
Electric Field Measurement: Measurement of electric fields generated by natural or induced currents in the ground.
Security Systems:
Real-Time Monitoring:
AI and Machine Learning: Implementation of AI and machine learning algorithms to continuously analyze incoming data and differentiate between friendly and hostile entities.
Secure Data Transmission: Use of encrypted channels to ensure the security of data being transmitted to command centers.
Intrusion Detection:
Automated Alerts: Systems that automatically alert security personnel to potential threats based on data analysis.
Integration with Defense Systems: Linkage of detection systems with automated defense mechanisms for rapid response.
Identification and Verification:
Biometric Sensors: Use of biometric sensors to confirm identities of detected individuals.
RFID and IoT Devices: Equipping friendly personnel with RFID tags or IoT devices for positive identification.
Scientific Principles:
Electromagnetic Wave Propagation:
Subsurface Scattering: Understanding and modeling how electromagnetic waves scatter when they encounter different materials underground.
Wave Attenuation: Studying how wave strength diminishes with distance and designing systems to counteract this effect.
Acoustic Wave Propagation:
Speed of Sound in Different Materials: Researching how sound waves travel through various underground materials to improve seismic imaging accuracy.
Echo Analysis: Techniques for analyzing echoes from seismic waves to create detailed underground maps.
Geophysical Surveying:
Resistivity Measurements: Techniques for measuring the electrical resistance of subsurface materials to detect anomalies.
Gravity Surveys: Use of gravimetric methods to detect density variations underground.
Mathematical Equations and Literacy:
Maxwell’s Equations:
Electromagnetic Fields: ∇⋅E=ρϵ0∇⋅E=ϵ0ρ, ∇⋅B=0∇⋅B=0, ∇×E=−∂B∂t∇×E=−∂t∂B, ∇×B=μ0J+μ0ϵ0∂E∂t∇×B=μ0J+μ0ϵ0∂t∂E.
These equations describe the behavior of electromagnetic fields in the subsurface environment.
Wave Equation:
Electromagnetic Waves: ∇2E−μϵ∂2E∂t2=0∇2E−μϵ∂t2∂2E=0 for modeling how radar waves propagate through the ground.
Acoustic Waves: ∇2u−1v2∂2u∂t2=0∇2u−v21∂t2∂2u=0 for seismic wave propagation.
Signal Processing:
Fourier Transform: F(ω)=∫−∞∞f(t)e−iωtdtF(ω)=∫−∞∞f(t)e−iωtdt for analyzing the frequency components of the received signals.
Kalman Filter: Algorithms for filtering and predicting the state of a system from noisy measurements.
Technological Innovations:
Advanced Sensors:
Multi-Spectral Imaging: Use of sensors that operate across multiple wavelengths to gather comprehensive data.
Quantum Sensors: Utilization of quantum technology for extremely high sensitivity and precision.
Data Fusion:
Integrated Platforms: Systems that combine data from GPR, seismic sensors, and magnetotellurics into a unified model.
3D Visualization: Advanced software for creating real-time 3D maps of underground structures and activities.
Autonomous Systems:
Drones and Robots: Deployment of autonomous drones and robots equipped with sensors to explore and map underground environments.
AI-Driven Analysis: Use of artificial intelligence to autonomously interpret data and identify patterns indicating friendlies or hostiles.
Conclusion:
The implementation of a system capable of seeing through the ground to any depth and identifying friendly or hostile actors requires an interdisciplinary approach. This includes the integration of advanced engineering, robust security measures, cutting-edge scientific research, sophisticated mathematical modeling, and innovative technology. This combination ensures the creation of a highly effective and precise underground surveillance and identification system.
=======================================
#(4):
"S.R.G./Iron Dome: Force Field tech";
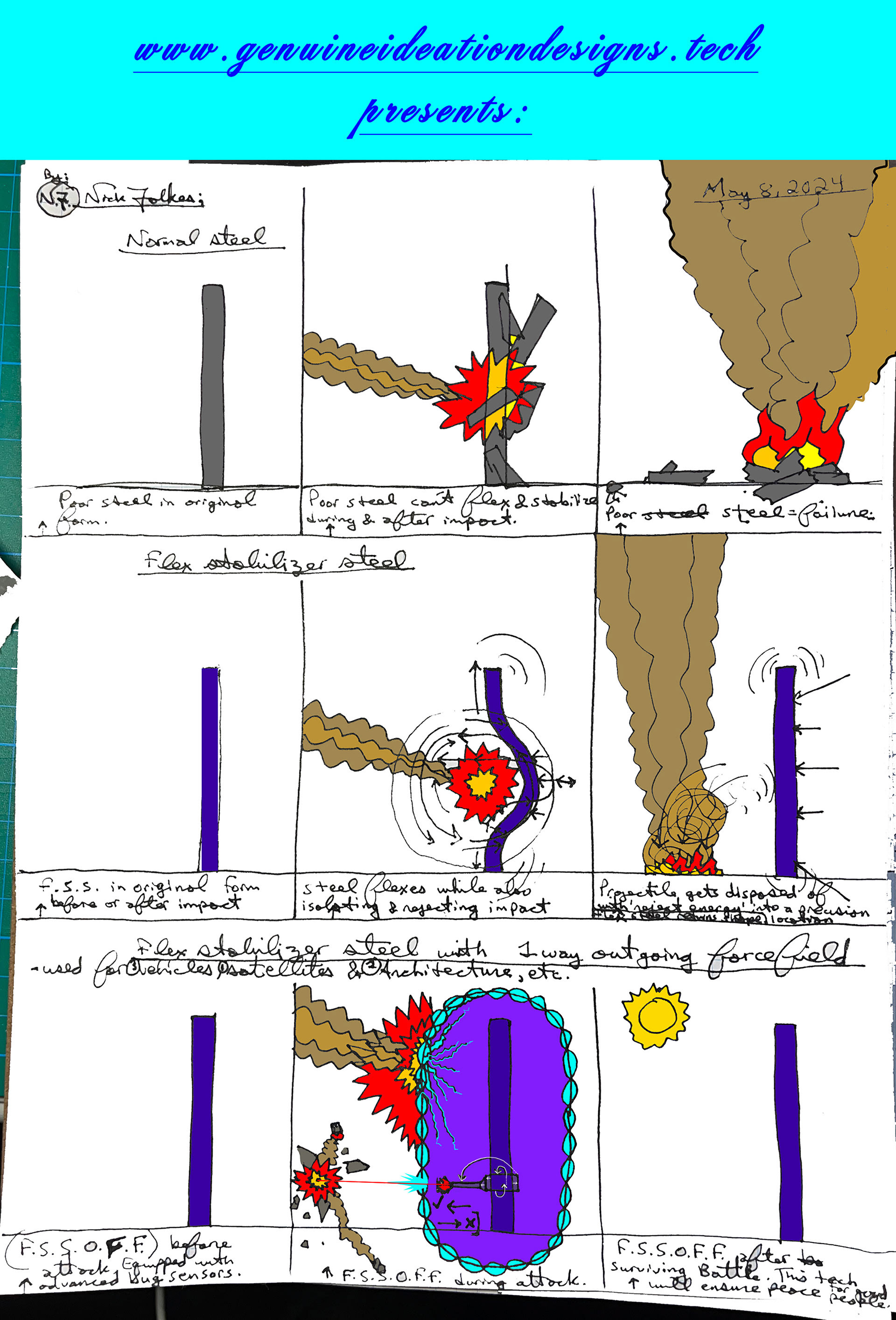
SRG force field:
what is the scientic mathematical equation to make a healthy electro magnetic defence force field that is programmable to be outgoing only?
Creating a scientific and mathematical equation for an electromagnetic defense force field that is programmable to be outgoing only involves complex physics and engineering concepts. Below is an outline of the theoretical principles and components required for such a system:
Fundamental Concepts
Maxwell's Equations: These are the foundation of classical electromagnetism, describing how electric and magnetic fields are generated and altered by each other and by charges and currents.
∇⋅E=ρϵ0(Gauss’s Law for Electricity)∇⋅B=0(Gauss’s Law for Magnetism)∇×E=−∂B∂t(Faraday’s Law of Induction)∇×B=μ0J+μ0ϵ0∂E∂t(Ampeˋre’s Circuital Law with Maxwell’s Addition)∇⋅E=ϵ0ρ(Gauss’s Law for Electricity)∇⋅B=0(Gauss’s Law for Magnetism)∇×E=−∂t∂B(Faraday’s Law of Induction)∇×B=μ0J+μ0ϵ0∂t∂E
(Ampeˋre’s Circuital Law with Maxwell’s Addition)
Lorentz Force Law: Describes the force experienced by a charge in an electromagnetic field.
F=q(E+v×B)F=q(E+v×B)
Plasma Physics: Plasmas can be used to create dynamic and programmable electromagnetic fields.
Programmable Outgoing Only Field
To create a force field that is outgoing only, we need to consider:
Field Generation: Using oscillating electric and magnetic fields to generate a force field.
E(t)=E0cos(ωt+ϕ)E(t)=E0cos(ωt+ϕ)B(t)=B0cos(ωt+ϕ)B(t)=B0cos(ωt+ϕ)
Directional Control: Ensuring the field interacts with objects only in one direction. This might involve using plasma mirrors or phased array antennas.
Eoutgoing=E0ei(ωt−k⋅r)Eoutgoing=E0ei(ωt−k⋅r)Boutgoing=B0ei(ωt−k⋅r)Boutgoing=B0ei(ωt−k⋅r)
Programmability: Controlling the field's properties via a computer program that adjusts the parameters of the field generators in real-time.
Mathematical Model
Let's consider a simplified model where we use a circularly polarized electromagnetic wave to create an outgoing field:
E=E0(x^cos(ωt−kz)+y^sin(ωt−kz))E=E0(x^cos(ωt−kz)+y^sin(ωt−kz))B=B0(y^cos(ωt−kz)−x^sin(ωt−kz))B=B0(y^cos(ωt−kz)−x^sin(ωt−kz))
Where:
E0E0 and B0B0 are the amplitudes of the electric and magnetic fields.
ωω is the angular frequency of the wave.
kk is the wave number, related to the wavelength λλ by k=2πλk=λ2π.
To ensure the field is outgoing only, boundary conditions must be imposed:
Reflecting Boundaries: Use materials or plasma mirrors that reflect incoming waves and only allow outgoing waves.
Phase Control: Adjust the phase of the wave generators to cancel incoming waves while reinforcing outgoing waves.
Implementation
Implementing this involves:
Designing antennas or field generators capable of producing the required electromagnetic waves.
Using sensors to detect incoming waves and dynamically adjust the field parameters to nullify them.
Employing a control system that processes sensor data and adjusts the field generators in real-time.
Conclusion
The exact implementation would require significant research and development, leveraging advanced technologies in electromagnetism, plasma physics, and control systems. While the above provides a theoretical framework, practical considerations and safety measures must be thoroughly addressed in a real-world application.
==============================================